The properties of materials are the basis of many applications; explaining them is also sometimes still a challenge for researchers. The most familiar physical property is the ability of a material to conduct (metals) or not (insulators) electric current. The conductivity of metals increases when the temperature decreases. Thus, copper conducts more than ten times better the current at -200°C than at 400°C. But for V2O3 (Vanadium III oxide), a metal at room temperature, the conductivity drops sharply by a factor of a million or more when the temperature falls below -113°C. It becomes an insulator at low temperatures! How to explain this astonishing transition from metal to insulator?
The mystery is even greater because, according to the conventional quantum model describing electrons in solids (Bloch's theory), the metallic or insulating character of a material depends only on its number of electrons. According to this model, electrons progressively fill states in regions of allowed energies (called energy bands) separated by regions of forbidden energies (gaps). If the last occupied energy band is partially filled, the material is metallic and the electrons are mobile. If it is completely filled, the material is insulating. The material can be compared to a stadium: if all the tickets have been sold, no spectators can move; if there are still empty seats, a "spectator flow" can be established. Since the number of electrons in a material does not change with temperature, a metal, according to Bloch, must remain so as it is cooled down!
What happens in V2O3 that challenges this well-tested model? The last partially occupied band is formed from orbitals fairly localized in space. The electrons cannot easily avoid each other and are subject to strong mutual repulsion, neglected in Bloch's model: the electronic correlations. At low temperatures, this repulsion would prevent the electrons from moving, making the compound an insulator. In our stadium, the match opposes two teams whose supporters hate each other. Massed in two opposite stands, separated by empty bleachers. Thus, even if the stadium is not full, the spectators do not move, in order to avoid interactions between fans.
The metal-insulator transition would thus be a manifestation of electronic correlations, but the microscopic processes accompanying it remain controversial despite 50 years of research. A clear observation of the evolution of the electronic energy bands during the transition was missing. Such a study can be performed with the Angle-Resolved PhotoEmission Spectroscopy (ARPES) technique, but for several important technical reasons was so-far missing. In the new experiments, carried out in part on the CASSIOPEE beamline, these problems were circumvented by working on a thin film of V2O3 deposited on a sapphire substrate.
The main result of this study, led by researchers at ISMO (Université Paris-Saclay), in collaboration with researchers from the University of San Diego (USA), Tohoku University and the KEK synchrotron (Japan), the CASSIOPEE bamline of SOLEIL, the LPS (Université Paris-Saclay), the IPP (Ecole Polytechnique), the LPEM (ESPCI), the University of Minas Gerais (Brazil) and the University of Twente (Netherlands), is presented in figure 1. The figure shows the evolution of the energy bands measured by ARPES during the metal-insulator transition by decreasing the temperature (panels A to F), or by increasing it (panels F to K). The transition is clearly observed on the measurements:
- At 180K (i.e. -93°C, red image), a parabolic-shaped energy band (typical of mobile electrons) intersects the "0" energy: the compound is metallic.
- At 100K (-173°C, dark blue image), there are no more electronic states in this energy zone: V2O3 has become insulating.
When the temperature decreases between 180K and 100K, the intensity of the parabolic band decreases gradually, while a horizontal band, typical of electrons remaining localized on the atomic sites, becomes more and more intense, indicating that mobile electrons "transmute" into localized electrons. Moreover, this horizontal band moves gradually towards higher binding energies. The trend is reversed by heating, but with a shift in temperature (compare the images at 120K and 130K by cooling and heating cycles), which can be explained by the formation, at intermediate temperatures, of metallic and insulating islands whose proportion depends on the previous state of the material.
This first imaging of the evolution of the electronic structure of V2O3 during its transition from metal to insulator thus allows to account for it as a gradual transfer of itinerant electron states to localized states. It shows that the metal-to-insulator transition involves a complete reorganization of several energy bands.
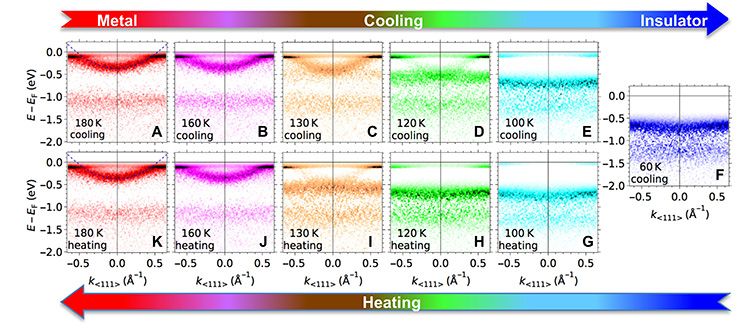
Figure 1: Evolution of the band structure of V2O3 measured by ARPES as a function of temperature through its metal-insulator transition, either decreasing the temperature (panels A to F) or increasing it (panels F to K).