The ability to control the magnetic properties of a material by means of an electric field represents a "Holy Grail" for applications in the field of magnetic memories. For this reason, so-called "multiferroic" compounds - with their coupled properties of P polarization (ferroelectricity) and M magnetization (magnetism) - are of great interest to researchers. This coupling results in the appearance of an excitation called "electro-magnon", measurable by absorption spectroscopy in the THz energy range (between infrared and microwaves) at very low temperatures.
Researchers from SOLEIL's AILES beamline, together with researchers from the University of Paris 7, have demonstrated such a P/M coupling in copper monoxide, CuO, using THz spectroscopy. Furthermore, extrapolation of experimental measurements shows that the electro-magnon measured could remain stable up to room temperature if high pressure is applied to the sample.
The concepts of electromagnetism arose from the idea of a link between electric and magnetic fields. Maxwell's equations, established in 1865 by James Clerk Maxwell, form the basis. The current barrier to the use of multiferroic materials in everyday applications lies in the low (cryogenic) temperatures required to stabilize multiferroic phases. At present, a great deal of research is aimed at achieving stabilization of multiferroic phases at room temperature. This study shows that it is possible to stabilize and even strengthen the electro-magnon in copper monoxide CuO down to room temperature by applying pressure to the sample.
To achieve this, a diamond anvil cell (DAC) was used: it enables hydrostatic pressure of a few GPa (approx. 10,000 to 40,000 atmospheres) to be applied by pressing the sample placed in a metal seal between 2 diamonds (see presentation photo). As diamond is a transparent material in the infrared (IR) and THz, it is possible to perform IR absorption spectroscopy measurements through this sample placed in the small volume inside the CED (cf fig 1).
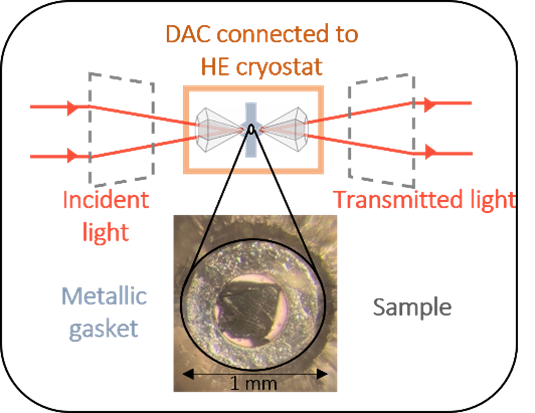
Figure 1: Schematic representation of the experimental set-up with a photo of the CuO sample in the diamond anvil cell.
Electromagnon is a low-intensity excitation, given the sub-millimeter dimensions of the sample; its measurement was nevertheless possible thanks to the high brilliance of the THz beam operated by the AILES beamline. Absorption spectra were measured at various pressures in the DAC (0.3 GPa, 1 GPa, 2 GPa and 3.3 GPa), from low temperature (170 K) to room temperature. Figure 2 shows the evolution of the electro-magnon's temperature range (maximum absorbance) as a function of pressure. It can be seen that, as the pressure in the cell increases, the electro-magnon exists over a wider temperature range (the spot of color, which corresponds to the electro-magnon, spreads out more and more towards low and high temperatures) and becomes stronger (the intensity of excitation increases, reflected by the color moving towards red).
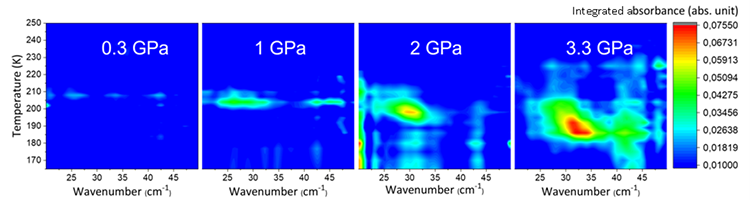
Figure 2: 2D map of THz absorbance spectra as a function of temperature for different pressure values in the CED (0.3 GPa is equivalent to almost 3000 times atmospheric pressure). At atmospheric pressure (measured outside the DAC) an electro-magnon is also measured, but over an even smaller temperature range than at 0.3 GPa.
Conclusion
When hydrostatic pressure is applied to a CuO sample, the strength of the electro-magnon, a characteristic excitation of the multiferroic phase, increases by an order of magnitude, and its temperature range expands by around 40 K. Extrapolation of these data predicts stabilization of the multiferroic phase at room temperature at a pressure of around 8 GPa (80,000 atm; see figure 3, red star).
Recent studies show that equivalent pressure values can be obtained by growing materials as thin films on a substrate that allows a stress to be applied that is more or less equivalent to that of hydrostatic pressure. This work paves the way for the use of multiferroic materials in electronic devices - devices that traditionally incorporate materials in the form of thin films.
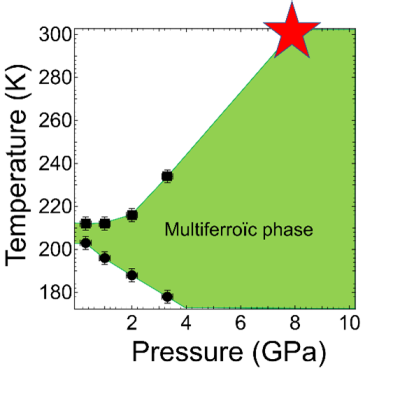
Figure 3: Pressure-temperature phase diagram of the CuO multiferroic phase obtained from the electro-magnon. The black dots are experimental measurements, and the red star is obtained by extrapolation.