Research involving the SOLEIL SEXTANTS beamline team together with researchers at UPMC, Université d'Orsay and the Italian synchrotron ELETTRA, show the potential of using linearly polarized X-rays for imaging magnetic domains in thin layers, as an alternative to circular polarization. According to these results, a very large efficiency gain can be envisaged in holographic imaging of magnetic domains using femtosecond X-ray sources (HHG and X-lasers) which, to date, produce linearly polarized X-rays only.
For imaging magnetic domains using X-ray holography, one tunes the photon energy at a core absorption resonance and relies on the magnetic circular dichroism effect: since the absorption coefficient depends on the sign of the magnetization, each domain distribution within the object produces a specific diagram in coherent scattering. The two-dimensional Fourier transform (2D-FT) will image the magnetic domain structure as dark/bright areas, according to the local projection of the magnetization along the beam axis.
X-ray holography is finding many applications in the field of ultrafast magnetization dynamics at femtosecond x-ray facilities, either HHG or 4th generation sources. Among many exceptional characteristics of these sources, there is up to now one limitation, i.e. the linear polarization state of the X-rays. Elliptical polarization is obtained by introducing a Faraday polarizer, a process whose overall efficiency, in the order of 1%, is unsatisfactory. For instance, a Co-based Faraday filter produces a circular polarization rate of about 30% at the maximum of the Co-L3 edge with a loss of 96% of the incoming intensity (values from the SXR station of LCLS, Stanford).
In the experiment described here, the scientists used the synchrotron radiation produced by a helical undulator to show that linearly polarized light can provide the same magnetic contrast as a 100% circularly polarized beam of the same intensity, suggesting that a gain of about 2 orders of magnitude in efficiency is possible.
First of all, they implemented at SOLEIL the so-called separate mask/sample mode, which allows one to bring any selected area of the sample into the field of view defined by the object aperture of the coherence mask. In this approach, the reference waves go through the sample, undergoing magnetic scattering. Then, they introduced a new method for imaging perpendicular magnetic domains using linearly polarized x-rays, which relies on Faraday rotation (real part of the index) rather than on circular dichroism (imaginary part). Since Faraday rotation is optimum at energies below the absorption maximum, there is also a gain in transmission efficiency. Fig. 3 shows that images of equivalent contrast can be obtained with either linearly or circularly polarized photons, the former requiring shorter acquisition times. This is important for future experiments at X-FEL and HHG sources, where circular polarization is not readily at hand.
One should stress the importance, for this study, of disposing of a source of selectable polarization, capable of producing a 100% polarized X-ray beam, either circular or linear. The helical undulator of the SEXTANTS beamline allowed us comparing the results obtained for the different polarizations quantitatively, therefore establishing the viability of magnetic imaging with linear polarization.
X-ray imaging by coherent scattering
This technique takes full advantage of all the most peculiar characteristics of advanced X-ray sources: high degree of coherence (for a large field of view), high flux and short pulse duration (for time resolution). Moreover, element selectivity and magnetic sensitivity are at hand when using energy- and polarization-tunable x-ray sources. X-ray imaging by Fourier transform holography (FTH) relies on the interference between coherent beams scattered by the object to be imaged and by one or more reference apertures (Fig. 1). When the sample is illuminated by a coherent wave-front, the spatial distribution of the optical constants within the object generates a unique angular distribution of the scattered wave and this information is coded in phase and amplitude at the detector level, via the interference with the reference waves (Fig. 2a). The Fourier transform of the two-dimensional scattering diagram (2D-FT) corresponds to the autocorrelation function of the entire sample (object+references). By an appropriate design of the coherence mask, the cross-correlation between object and references can be well separated from the self-correlation of each one of them (Fig. 2b), providing an image of the object with a resolution limited primarily by the diameter of the reference aperture (Fig. 2c).
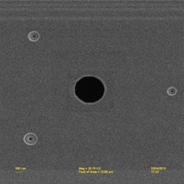
Figure 1: SEM image of a coherence mask featuring a 2 µm circular object aperture and three reference apertures placed 5 µm away. The opaque mask is formed by 1 µm of Au deposited on a Si3N4 substrate (F. Fortuna, CSNSM Orsay).
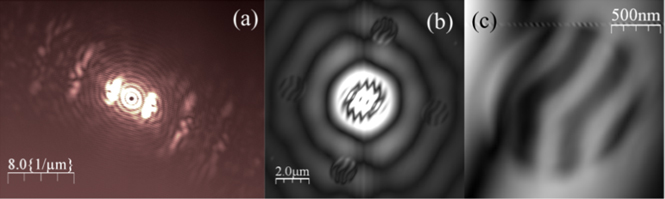
Figure 2: Co/Pd) magnetic multilayer. (a) Scattering diagram collected using 777eV linearly polarized photons. (b) Selected area of the FT showing the sample autocorrelation, with two independent reference-object cross-correlation images. (c) Zoom on the right-side cross-correlation image.
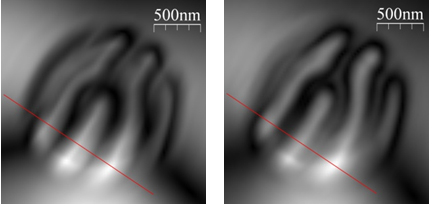
Figure 3: Holographic images of magnetic domains in a (CoPd) multilayer collected using 100% linearly polarized x-rays at 777eV (left) and 100% circularly polarized x-rays at 778eV (right).