A challenge for further development of Li-ion batteries, especially for new applications requiring more energy, like electric vehicles or storage of renewable energies, is the design of new positive electrode materials with higher massic capacities, i.e. able to exchange more Li ions for a same material weight. For these materials, the presence of a transition metal is necessary to reach the required high electrochemical potentials. This is, however, to the detriment of the massic capacity because transition metals have a greater molar mass than light elements like carbon or oxygen.
A possible solution consists in materials able to exchange more Li+ ions due to redox reactions involving not only the cation (the metal), like in classical materials, but also the anion. But these so-called "Lithium-rich" materials of general formula Li1+xM1-yO2 (where M is a transition metal or a mixture of several transition metals) pose numerous issues: redox processes involving oxygen (the anion) lead to irreversible structural rearrangements, or even gaseous oxygen release, with detrimental consequences on electrochemical properties: massic capacity fading upon charge/discharge cycles, sluggish kinetics and voltage fading. Such problems are due to a mismatch between metal nd electronic levels (3d for usual metals) and anion np levels (2p for oxygen).
Researchers from the "Solid state chemistry and Energy" group of Collège de France, Paris, decided to work on anions located in the same column as oxygen in the periodic classification of elements (chalcogens), namely sulfur (S) and selenium (Se), allowing a better interaction between anion 3p or 4p levels and metal 3d levels. Thanks to a collaboration with University of Pau & Pays de l'Adour (IPREM, E2S-UPPA) and SOLEIL GALAXIES beamline, they could evidence by HAXPES (Hard X-ray Photoemission Spectroscopy) the complex redox processes involving the metal and S2- and Se2- anions in Li2TiS2.4Se0.6 material, by analyzing photoelectrons coming from the electrodes during irradiation by very high energy photons (10 keV).
The use of 10 keV photons at GALAXIES beamline, with respect to standard energy photons (1487 eV), presents three advantages:
(1) multiply by 4 the Se 3p/S 2p intensity ratio, highlighting the Se 3p signal of selenium, which is usually difficult to exploit due to overlapping with S 2p signal of sulfur,
(2) access the S 1s signal of sulfur (high energy signal), which does not overlap with any selenium signal, allowing thus an easy interpretation of redox phenomena of sulfur,
(3) significantly increase the probing depth of photoemission (> 40 nm) in order to get rid from parasitic signals coming from the material's surface, always present because the electrode shows a parasitic interfacial reactivity with the electrolyte in a lithium battery.
Figure 1a shows electrochemical charge/discharge curve and the potential increase/decrease when lithium is extracted/reinserted into the material. The different points correspond to the different HAXPES spectra (figure 1b, 1c) in order to reveal redox processes involving sulfur and selenium. Although those two elements are both oxidized upon charge (their negative charge decreases from 2- to n-, n<2) they show different behaviours. The oxidation of selenium is observed earlier and is deeper than for sulfur. This decoupling between S and Se behaviours explains why the electrochemical performance of a material using those two elements is much better than a material using only sulfur (Li2TiS3) or selenium (Li2TiSe3).
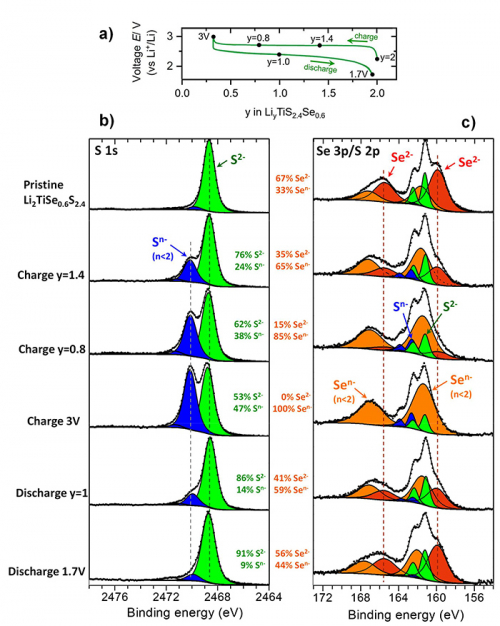
Figure 1:
a) Charge/discharge curve of Li2TiS2.4Se0.6 material;
b, c) HAXPES S 1s, 2p and Se 3p spectra at 10 keV showing gradual oxidation / reduction the anions upon charge / discharge. Se is earlier and more deeply oxidized than S.
This study shows that independent anionic activities can coexist within the same compound and offer new opportunities to optimize positioning between ligand np energy levels and metal nd energy levels in order to improve the electrochemical performances of transition metal chalcogenides as positive electrode materials for lithium-ion batteries.