When a magnetic material is shined on with a laser pulse, it will locally demagnetize. This magnetization loss occurs in an ultra-fast timescale, here less than a picosecond (10-12 s). In some exceptional cases, the material can even undergo a global magnetization reversal. In addition to the theoretical importance of this phenomenon, this new "all-optical" control of the magnetization of materials paves the way toward a new generation of electronic and magnetic devices, particularly in data storage and processing technologies.
Researchers from the Institut Jean Lamour in Nancy and the SOLEIL CASSIOPEE beamline have studied the phenomenon of ultra-fast demagnetization in Heusler compounds Co2MnAlxSi1-x, to shed light on the still poorly understood mechanisms at the origin of this light-matter interaction.
In this study, thin monocrystalline layers of Co2MnAlxSi1-x Heusler compounds (see https://en.wikipedia.org/wiki/Heusler_compound) are elaborated using molecular beam epitaxy. Firstly, the thin layers structure is carefully analyzed using electron diffraction, X-ray diffraction, and transmission electron microscopy at the Institut Jean Lamour. Even though a B2 type chemical disorder (Mn/Si/Al mixture) is obtained when substituting Si by Al (figure 1-left), the high crystalline quality together with the Heusler structure are preserved for the entire substitution range.
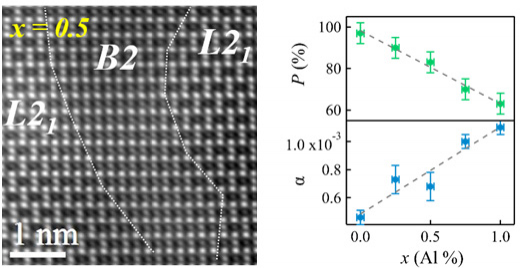
Figure 1: Left: transmission electron microscopy image of Co2MnAl0.5Si0.5 compound, we can see B2 disordered areas (Mn/Si/Al mixture) and L21 ordered areas. Right: spin polarization (P) measurements of the Co2MnAlxSi1-x series obtained on CASSIOPEE and the corresponding Gilbert damping α measured at the Institut Jean Lamour.
This series of compounds is then elaborated and studied on the SOLEIL CASSIOPEE beamline, where the spin polarization P of the electronic states near the Fermi energy can be measured using in-situ spin resolved photoemission spectroscopy.
As shown in figure 1-right (green curve), P varies linearly from Co2MnSi which is a half-metal magnet (100% spin polarization) to Co2MnAl which is a standard ferromagnet (63% spin polarization). This series of compounds is therefore an excellent playground to study the impact of the electronic structure at the Fermi energy on the ultra-fast magnetization dynamics. In addition, the study of the magnetization precession around its equilibrium position, which plays a key role in the current-induced manipulation of the magnetization , is performed at the Institut Jean Lamour by using Ferromagnetic Resonance. The ease with which the magnetization can be put into a precession motion is described by a dimensionless constant: the Gilbert damping α. The lower this damping, the longer the magnetization precession and the lower the energy cost. Gilbert damping values of the whole range of substitution are reported in figure 1-right (blue curve). It is inversely proportional to the spin polarization, thereby confirming the theoretical models on the relationship between those two quantities and in particular, confirming the ultra-low damping values in half-metal magnets. The study of this magnetization dynamics, which is not "ultra-fast" (the timescale here is in the nanosecond order of magnitude, 10-9 s) is intended to explore the possible links between α and the ultra-fast demagnetization time.
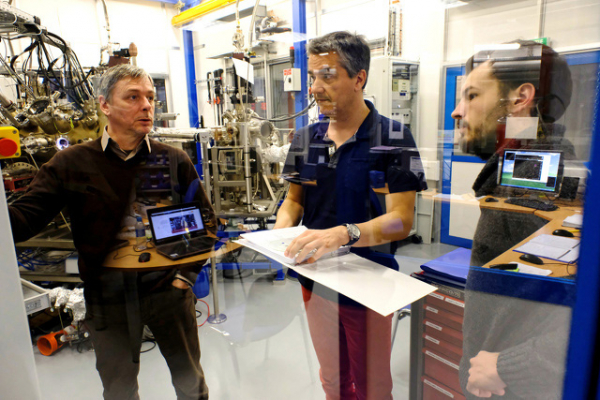
From left to right: Stéphane Andrieu, François Bertran and Charles Guillemard, at CASSIOPEE beamline.
Ultrafast magnetization dynamics measurements were obtained at the Institut Jean Lamour by mean of Time-Resolved Magneto-Optical Kerr Effect -TR-MOKE- with a pump-probe geometry. The demagnetization curves of Co2MnAlxSi1-x compounds are shown in figure 2-a. The scientists observed that the samples loose approximately 15% of their magnetization just after the first laser pulse is delivered, at t0 = 0 ps . In addition, the characteristic demagnetization time τM varies significantly with the substitution rate x, plotted in figure 2-b. It decreases from τM = 380 fs for Co2MnSi to 165 fs for Co2MnAl. Finally, in figure 2-c, the demagnetization time τM is observed to be inversely proportional to 1-P. This experimental dependence is of great interest to test theoretical models.
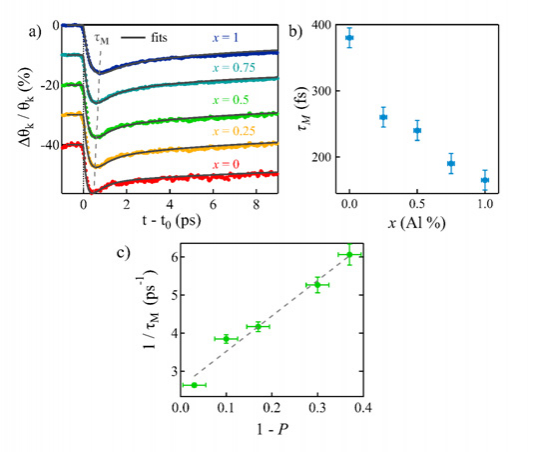
Figure 2: a) Demagnetization curves obtained by TR-MOKE on the Co2MnAlxSi1-x series as a function of the delay time, b) Characteristic demagnetization time τM as a function of Al rate and c) τM plotted as a function of 1 – P.
Even though demagnetization times are predicted to be longer in half-metal magnets, the trend in Figure 2-c is in excellent qualitative agreement with some theoretical models. In these models, the demagnetization time is inversely proportional to the amount of scattering mechanisms of the electrons spin momentum. This spin scattering is significantly reduced in half-metal magnets such as Co2MnSi due to the spin gap in the density of states, which makes the demagnetization time much longer than in a standard ferromagnet like Co2MnAl.
In summary, it was first demonstrated that the spin polarization of Co2MnAlxSi1-x compounds can be tuned according to the Al substitution rate x. Moreover, even if the spin scattering mechanisms responsible for the Gilbert damping and ultrafast demagnetization may be different, it appears that the spin polarization of electronic states near the Fermi energy plays a major role in the magnetization dynamics, whether at the nanosecond or picosecond timescale.