Nuclear fusion, the reaction that powers the Sun and stars, could be a solution for an abundant and CO2-free industrial source of energy on Earth. However, some scientific and technological issues have yet to be solved.
The objective of Tokamak ITER, thanks to the fusion of Deuterium ("D") and Tritium1 ("T") nuclei, is to produce 400 MW over a significant period of time (~16 minutes). To succeed in producing enough fusion reactions from these nuclei during this short time would be a first step towards more realistic fusion reactor (DEMO).
Within the framework of the EUROFusion consortium bringing together scientists from Grenoble, Paris, Orléans, Patras, Eindhoven and Moscow, the DESIRS beamline participates in fundamental studies on one of the stages of fusion plasma heating: the production of hydrogen and deuterium negative ions.
In tonne of oil equivalent (toe), a unit of energy measurement, one tonne of wood is equivalent to 0.3 toe, one tonne of coal to 0.7 toe, while one tonne of uranium U235 is equivalent to 13 000 toe and one tonne of deuterium (D2) and tritium (T2), to 8 million toe! These examples clearly show why so much efforts are engaged in order to understand and control the (D-T) fusion process.
The ignition of these fusion reactions in tokamak plasma requires additional heating devices, in order to reach the threshold of these nuclear reactions: the plasma is heated to over 200 million degrees (ionic temperature Ti~20 keV) by radio-frequency waves and by Neutral Beam Injection (NBI), using a high energy and high power atomic deuterium beam (34 MW of 1 MeV D° on ITER). The ITER heating neutral system consists in accelerating Deuterium D- negative ions to 1 MeV and neutralizing them by stripping the additional electron so that the neutral atoms could penetrate the fusion plasma without being deflected by the magnetic field of the plasma cage. The neutrals in the plasma are re-ionized by collisions, then trapped by the confining magnetic field and therefore transmit their kinetic energy; it is a very effective heating process, essential for the ignition and control of fusion reactions.
For ITER, the initial negative ion beam is produced in a cold plasma (electron temperature Te < 1 eV) when deuterium atoms and/or ions collide or come close to the wall surface coated with caesium (Cs). To reach the negative ion density required for the ITER accelerator (JD-~250 Amperes per m2), Cs consumption is relatively high (~10 µg/s), this requires significant maintenance during ITER operation, Cs is highly reactive and its progressive diffusion into the accelerator stage can induce parasitic beams.
To overcome these obstacles, in the framework of the EUROFusion consortium, scientists from Grenoble, Paris, Orléans, Patras (Greece), Eindhoven and Moscow are conducting fundamental studies on the production of (H-/D-) 2 negative ions without the use of Cs in the development of the DEMO reactor.
The advantage of the DESIRS beamline
An alternative to the use of Cs could be the production of these (H-/D-) negative ions by dissociative attachment (DA) (see. Fig. 1) of ro-vibrationally excited molecules H2*(v’’,J")/D2*(v’’,J") in their electronic ground state (v" and J" indicate the vibrational and rotational state of the molecule) with cold electrons. These high ro-vibrational levels of molecules are essential for the DA mechanism: the effective reaction section increases by a factor of 10 000 between the v”=0 and v”=5 levels.
The absorption spectroscopy technique performed on the DESIRS beamline offers a unique method to directly probe these molecular levels produced by recombinative desorption on surfaces and by vibrational excitation (E-V reaction; see Figure 1) in the plasma volume.
The purpose of the work conducted on DESIRS is to compare the behaviour of several materials and their capacity to produce ro-vibrationally excited molecules. Several steps have already been taken on branch B of the DESIRS beamline:
- in 2016, first detection of the H2*(v”=2,J") level obtained with tungsten heated filaments;
- first detection of the H2*(v”=6,J") level in ECR hydrogen plasma3 and first demonstration of the effect of a material on the production of H2*(v”,J") molecules in 2017;
- first detection of H2*(v”=7,J") and D2*(v”=3,J") levels in ECR plasmas in 2018.
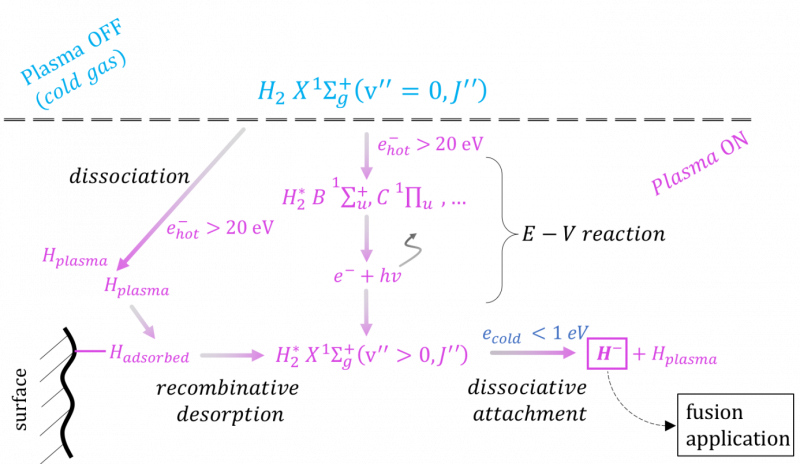
Fig. 1 From top: in a cold gas, without plasma, only the rotational levels j'' of the ground level v''=0 are populated. Hot electrons (Te > 20 eV) are produced in the plasma. From the left of the figure: recombinative desorption is a reaction between a hydrogen atom already adsorbed on the surface of the material and another atom from the plasma. The two atoms combine to form a molecule in a ro-vibrationally excited ground electronic state (v’’ >0). The dissociative attachment with a cold electron (Te < 1 eV) produces a negative ion; collisions between molecules and hot electrons produce singlet states (B and C) that radiatively de-excite into these same ro-vibrationally excited molecules. These mechanisms are identical for deuterium.
During these various experiments on the DESIRS beamline, several experimental conditions were explored: operating pressure, microwave power, wall temperature, position in the plasma volume and different materials. The use of Fourier Transform Spectroscopy (FTS) in December 2018 coupled to windowless set-up resulted in a significant improvement in both the wavelength range covered and the spectral resolution achieved; nine different settings were required to cover transitions from v’’=0 to v’’= 8 for H2 in the wavelength range from 105 to 165 nm, with a very high resolution (0.95 pm of FWHM4, i.e. resolving powers in the range of 150000). Effective data collecting (multiplex) on the
Fourier Transform branch enables a large number of parameters to be varied in a short period of time, which could only help understanding such a complex environment. Thanks to the exploitation of FTS spectra, it is possible to determine the absolute ro-vibrational distribution of H2 and D2 and so attempt to improve the production of these molecules in their excited states. For example, it will be possible to compare various materials, such as tantalum, tungsten, stainless steel or quartz, the latter is already well known for its very low recombination coefficient.
Figure 2 displays a typical absorption spectra obtained in D2 plasma facing a tantalum surface. On this rotational spectrum, the P and R branches are observed for transitions 0-6 up to J'' = 8. P and R transitions are used to determine the vibrational populations of the ground state to which the DA mechanism is sensitive.
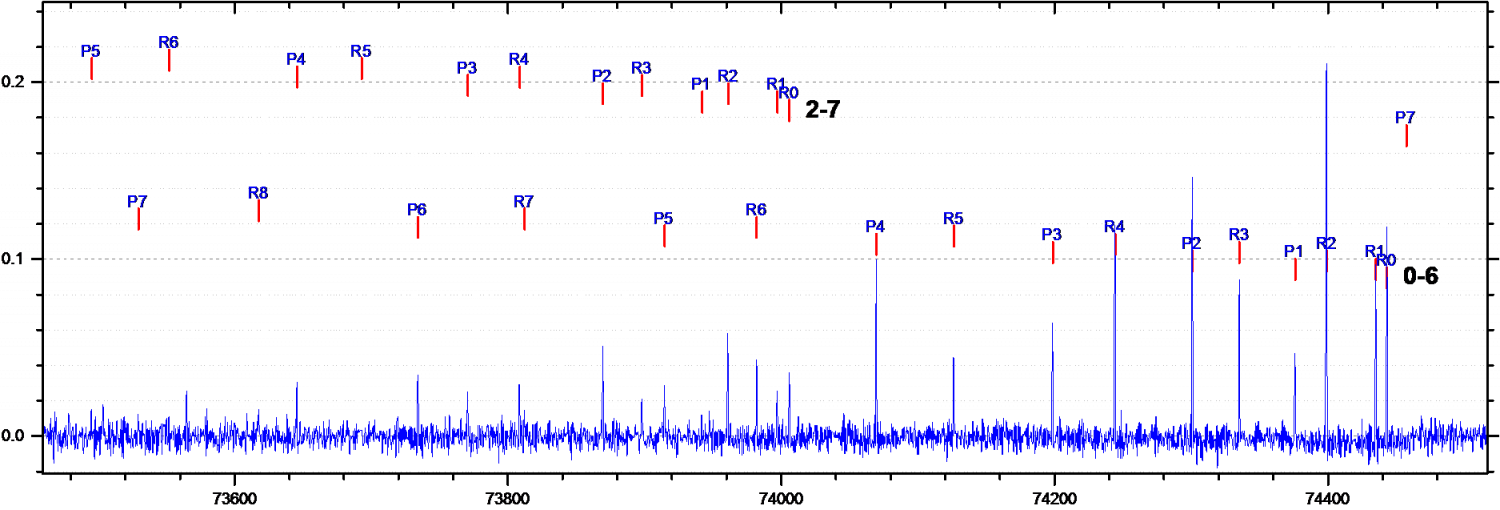
Figure 2: Absorbance (ln(Io/I)) of D2*(v"=6,J") as a function of the wave number (cm-1) (cm-1) obtained at 150W, 6 mTorr, for tantalum as a plasma-facing material. 0-6 and 2-7 correspond to transitions between two vibrational states of the molecule: from H2 (X 1Σ+g, v''= 6,J'') to state B 1Σ+u (v'= 0) and between H2 (X 1Σ+g, v''= 7,J'') and B 1Σ+u (v'= 2) . Io is the intensity of light from the synchrotron without absorption (reference) and I is the intensity of the light after absorption by the medium.
Moreover, in addition to the molecular transitions, the Lyman series of atomic transitions5 (see. fig. 3), from Ly-γ to Ly-θ (97.25 nm to 92.3 nm) were studied during the FTS measurements to obtain the absolute density of the hydrogen atoms and determine the absolute density of the different vibrational levels of the molecule in its ground state.
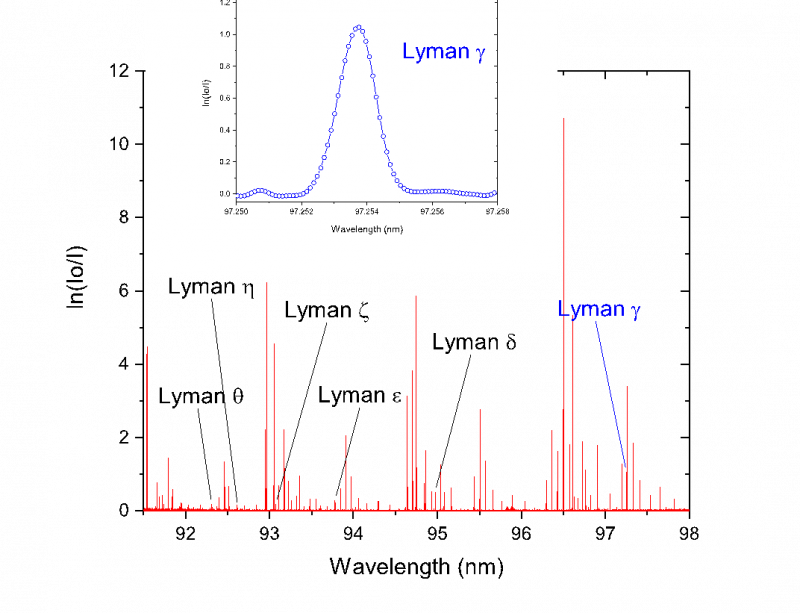
Fig. 3The absorption of Lyman atomic transitions obtained in H2 plasma for quartz as a plasma-facing material at low pressure and temperature (0.6 mTorr and 173 K) for a microwave power of 150 W. The Lyman series are used to obtain the absolute density of H or D atoms in plasma.
Outlook
Thanks to the development of this method for the diagnosis of H2/D2 plasma, it is now possible to perform spatially resolved absorption measurements of excited ro-vibrational levels of H2/D2 molecules in plasma. This study should allow the experimental validation of two-dimensional models before the design of new ion sources for fusion.
(1) Deuterium (D) and Tritium (T): very light atoms, both isotopes of the hydrogen atom (H): like H, their nucleus has one proton, but they also contain neutrons: one for D, two for T.
(2) The dihydrogen and H- ions will be used for the start-up of the ITER reactor and for its first years of operation before the use of molecular deuterium and D- ions. Molecular deuterium shall be used when nuclear fusion reactions are initiated in a second step.
(3) ECR: Electron cyclotron resonance, coupling mechanism between the electrical component E(t) of an electromagnetic frequency wave and the movement of an electron in a constant perpendicular magnetic field, used to produce the plasma.
(4) Parameter of the FTS linked to resolving power of the instrument . This is basically the minimum linewidth than can be clearly measured in a spectrum (FWHM stands for Full Width at Half Maximum). Obviously, if the value is small enough, the instrument could potentially measure narrow absorption features in a spectrum, which is frequently the case with atomic species or small molecules.
(5) The Lyman series https://fr.wikipedia.org/wiki/S%C3%A9rie_de_Lyman