Temperature plays a critical role in regulating body mechanisms. Local temperature increments above 42 °C are shown to kill cancer cells in tumorous tissue, leading to the development of nanoparticle-mediated thermo-therapeutic strategies. Hence, monitoring temperature changes within the core of nanoparticles, using nanothermometry, is gaining prominence in research.
A label-free, direct, and universal nanoscale thermometry technique shows promise in detecting substantial nanothermal fluctuations in tumor-mimicking tissues. It can effectively transform any material into a nanothermometer within biological environments. X-ray absorption spectroscopy, conducted at SAMBA beamline, was used to track thermal processes during photoexcitation.
Hyperthermia is an anticancer treatment that aims to elevate temperatures within tumors to eliminate cancer cells, with a particular emphasis on localized application to spare healthy tissues. Local temperature monitoring during hyperthermia treatments is essential to avoid undesired cytotoxic effects and to preserve healthy tissues. At the nanoscale, nanoparticles can function as nanoheaters, providing precise control over cell damage and facilitating targeted drug release.
Enhancing the heat capacity of nanoparticles is essential for precise therapeutic temperature control, as localized heating can be therapeutically effective without causing significant macroscopic temperature changes. However, measuring the nanoscale temperature within the core of nanoparticles remains a challenge, with many of the nanothermometry methods relying on indirect approaches.
Hence, the methodology presented here is based on a direct measurement approach: nanoparticles in tumor cells are heated with near-infrared (NIR) light and the local temperature is monitored following the temperature dependent atomic parameters of the nanoparticles with the use of X-ray absorption spectroscopy, specifically in the EXAFS regime. The measurements were conducted at SAMBA beamline at SOLEIL synchrotron due to its high beam stability, high-quality instrumentation and versatility. A schematic of the experimental setup is displayed in Figure 1A.
This research utilized nanoparticles of gold and iron oxide combined together (Figure 1B). These nanohybrids were chosen since they can be heated with NIR light and remain stable when internalized in an in vitro 3D tumor model of glioblastoma. The tumor model, called spheroid (a spherical self-assembled aggregate of glioblastoma cells), provides a realistic representation of an in vivo scenario (Figures 1B and 1D).
What makes this study groundbreaking is the possibility to directly measure the temperature of the nanoparticles under NIR photo-excitation inside tumor-mimicking tissues without the need for additional chemical elements or markers, one just needs to follow the evolution of the EXAFS spectra for the Au element at different temperatures (Figure 1C). It essentially functions as a universal nanoscale thermometer: gold has been 'tracked' in this work, but it could be another chemical element. Moreover, it also operates effectively across a wide temperature range, making it a versatile tool for studying thermal fluctuations (see Figure 1C).
This advanced technology can be applied to various nanomaterials for addressing biomedical challenges in cellular settings. The adaptability and versatility of EXAFS nanothermometry make it suitable for implementation in non-biological applications as well. For example, it can be employed for temperature monitoring of chemical elements in microelectronics, catalysis, fuel cells, or in the development of thermochromic materials.
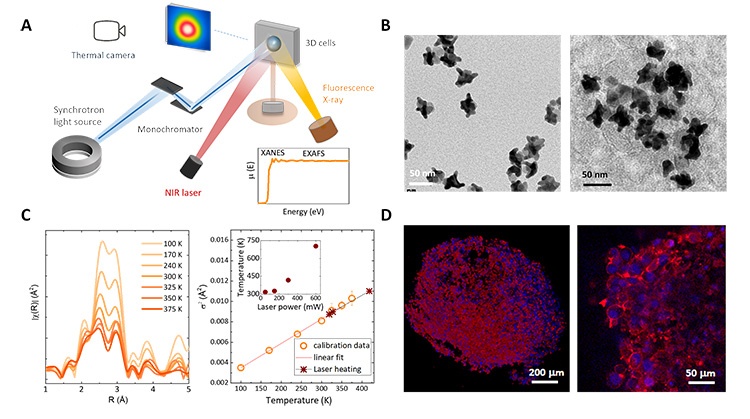
Figure 1:
A) Scheme of the experimental setup for acquiring X-ray absorption nanothermal spectroscopy inside cells, simultaneously applying X-ray and NIR laser irradiation to nanoheaters within 3D tumor spheroids.
B) Transmission electron microscopy images of synthesized gold-iron oxide nanohybrids and internalized in glioblastoma cells.
C) Fourier-transformed EXAFS signal in the calibration range (100-375 K) and temperature-dependent curve of Debye–Waller factor () (calibration fit curve) (open symbols). The solid symbols correspond to calculated values under photothermal excitation. Inset: Local temperatures attained under NIR laser excitation.
D) Confocal images of a labeled (with nanohybrids) spheroid – in red: cytoskeleton (it gives the cells constituting the spheroid their shape); in blue: cell nuclei (contain their DNA).