Over the last 20 years, colloidal nanocrystals have excited the interest of researchers because of their luminescence properties or, more recently, their use as a light source for displays. However, these nanocrystals are also very promising for optoelectronics, because they combine the robustness of inorganic materials with the ease of processing of organic materials.
The INSP team is interested in the (photo)-conduction properties of colloidal quantum well films of HgTe for the design of low cost and fast devices. Their results are published in NanoLetters.
Colloidal nanocrystals are semiconductor nanoparticles whose optical properties are tunable from the UV to THz range. For optoelectronics purpose, they are of particular interest for in the infrared range of wavelength, where existing technologies remain expensive and complex. However, for such wavelengths, investigating the dynamics of the charge carriers within these materials is a major challenge because the conventional techniques based on time-resolved optical measurements are difficult to implement. This is why the authors have used alternative techniques based on time-resolved photoemission on the TEMPO beamline and photoconduction.
Why study HgTe?
To address infrared wavelengths, narrow band gap materials are necessary. HgTe is an ideal candidate because it is a semi-metal in its bulk form and the excitonic transition observed in the nanocrystals of HgTe therefore results from a pure quantum confinement effect.
In this study, the authors used colloidal quantum wells (nanoplatelets) of HgTe recently synthesised by the group of Sandrine Ithurria at ESPCI in Paris. The 2D geometry leads to a perfect control of the thickness down to the single atom level and generates excitonic properties with no inhomogeneous broadening (i.e. the ensemble spectrum is the same as that of an individual particle). The challenge then is to make these materials conducting and photoconducting. This can be achieved by modifying the surface chemistry of the nanoplatelets. The authors have demonstrated that these changes not only increase the inter-particle coupling but also tuning the charge carrier density. To do this, the nanoplatelets are integrated in electrolytic transistors (Figure 1) and the material can either be a conductor of electrons (n-type) or holes (p-type) dependent on the surface chemistry used.
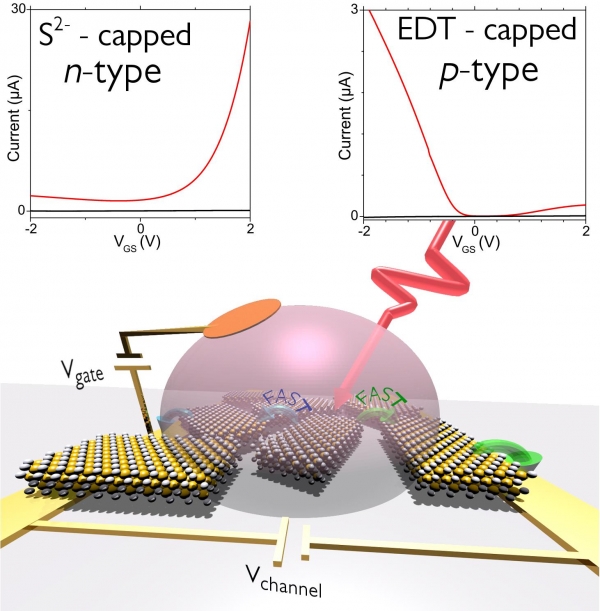
Figure 1: Top: transfer curves of transistors based on HgTe nanoplatelets, with S2- as the surface chemistry (left) or ethanedithiol (right). Bottom: schematic diagram of the transistor in which transport takes place by hopping between nearest neighbours.
The contribution of time-resolved photoemission
The authors have moreover shown that the photoconduction properties are driven by the surface chemistry of the nanoplatelets. The p-type material is a much better photoconductor thanks to its Fermi level close to the middle of the band gap. The response time of these transistors under illumination is of the order of 100µs to 1ms. It was then necessary to understand if this dynamic was an intrinsic property of the material or by contrast was linked to some device parameters.
To do this, the authors used time-resolved photoemission on the TEMPO de SOLEIL beamline. Figure 2 is a schematic diagram of the experiment. This instrument gives access to the relaxation time of the majority and minority carriers. The typical times obtained are in the range of 100ns to 1µs (Figure 2, bottom) dependent on the surface chemistry used, which suggests that the current response times of the photo-transistors may still be reduced by three orders of magnitude by adapting the geometry of the devices.
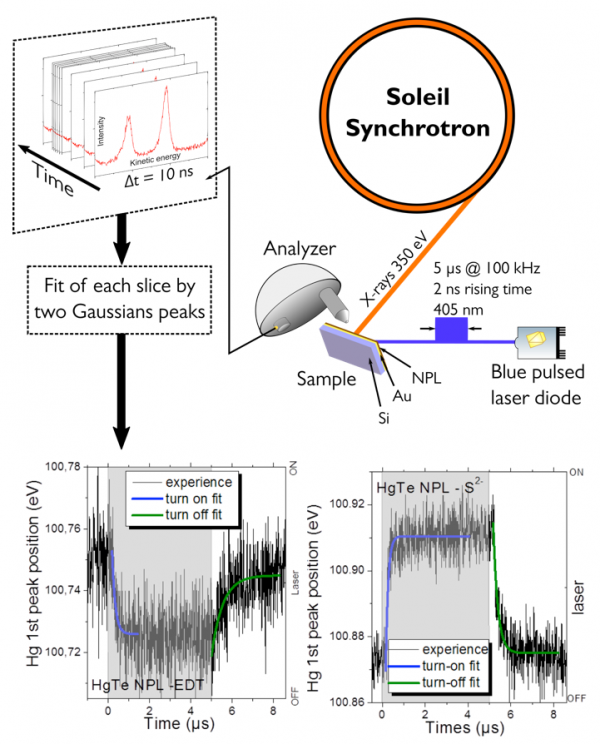
Figure 2: Top: schematic diagram of the time-resolved photoemission used to determine the relaxation time of the majority and minority carriers in the colloidal quantum wells of HgTe. Bottom: relaxation of the binding energy as a function of time of the Hg 4f state in response to a light pulse
The next challenge is now to extend this method to materials with an even narrower gap having optical properties in the mid to far infrared.