The transport of metallic trace elements (MTE), whether of natural origin or related to the human activities, is a major challenge in the preservation of ecosystems and public health. This transport is controlled by physical, chemical and biological processes that must be understood in order to anticipate and control possible environmental contamination.
In order to determine the fate of these MTE, why they are present and how they "travel", the Nano-BioGeochemistry team of the Geosciences Rennes laboratory have teamed up with the SOLEIL LUCIA beamline and the Interdisciplinary Laboratory for Continental Environments in Nancy. They sought to highlight the factors influencing the transfer of MTE in riparian* wetlands, whose seasonal flooding and drying up can cause drastic changes in physicochemical conditions, consequently giving them a major role in MTE mobility in the environment.
In certain regions of the world, the hydrological regime and the chemical composition of their waters are intimately linked to the bordering wetlands (marshes, lagoons, swamps, peat bogs, etc.). These zones play a buffer role that not only receives but also transforms and stores organic or inorganic chemical elements of natural or anthropogenic source. The riparian wetland is characterized by alternating water conditions. During the high-water level period, it is waterlogged and the river then plays a drainage role. During periods of low water level, it constitutes a water reserve that supports the water level in the river and groundwater. These particular conditions control the biogeochemical processes responsible for MTE mobility in these environments.
When soils are saturated with water, they gradually end up in anoxic conditions. Fe (III) phases and all associated elements, such as MTE, are then solubilized. Depending on the flow (lateral and vertical), the reduced water then loaded with Fe (II) and MTE come into contact with the river beds and/or the oxidizing water at the riverbanks. This causes Fe (II) oxidation to precipitate as solid Fe (III) at the riverbank surface due to the high oxygen content (Figure 1).
*riparian: relating to wetlands adjacent to rivers and streams
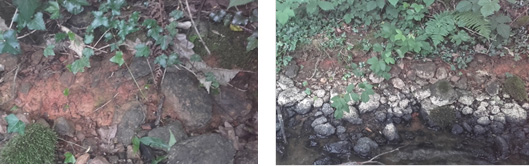
Figure 1: Ferric deposits observed in riverbanks of a river draining a wetland (Brittany, France).
These ferric deposits have properties favorable to the trapping of a large number of chemical elements. They therefore could play a geochemical filter role between the wetland and the river.
LUCIA analysis
The Fe K-edge X-ray Absorption Near Edge Structure (XANES) spectra were collected on the LUCIA beamline in order to characterize these ferric deposits observed on the surface. Iron speciation is mainly in the form of ferrihydrite (amorphous Fe oxyhydroxide), goethite (α-FeOOH, crystallized Fe oxyhydroxide, product of the transformation of ferrihydrite) and small Fe clusters associated with organic matter. Iron speciation appears different than those observed in subsurface of riverbanks where Fe occurs mainly in clays and goethite structures (Figure 2).
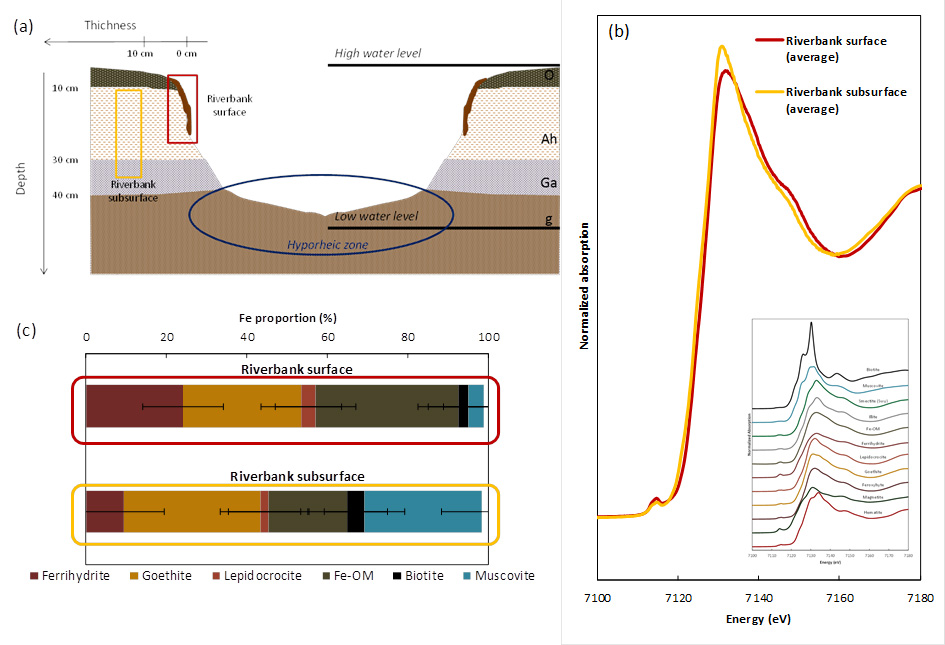
Figure 2: (a) Sketched cross-section of the riverbanks where ferric deposits are observed. (b) Fe K-edge XANES spectra for riverbank, surface and subsurface samples as well as the spectra of the reference compounds (clay, oxide, oxyhydroxide, organic matter-bound Fe) used to calculate the different Fe-bearing phases in the riverbank samples (c).
The difference in Fe speciation at this interface is essential for the mobility of the MTE since all the Fe present on these banks does not have the same properties. Certainly, due to their very small size and low crystallinity, ferrihydrite (amorphous iron oxyhydroxide) and Fe-organic matter associations are the main contributors to the sorption of chemical elements, here they are referred to as reactive Fe. A linear correlation exists between the reactive Fe content and the total concentrations of most MTEs. Riverbank surface samples have the highest levels of reactive Fe content and associated metals. Two representative examples of metal behavior are given in Figure 3: arsenic (As) and copper (Cu).
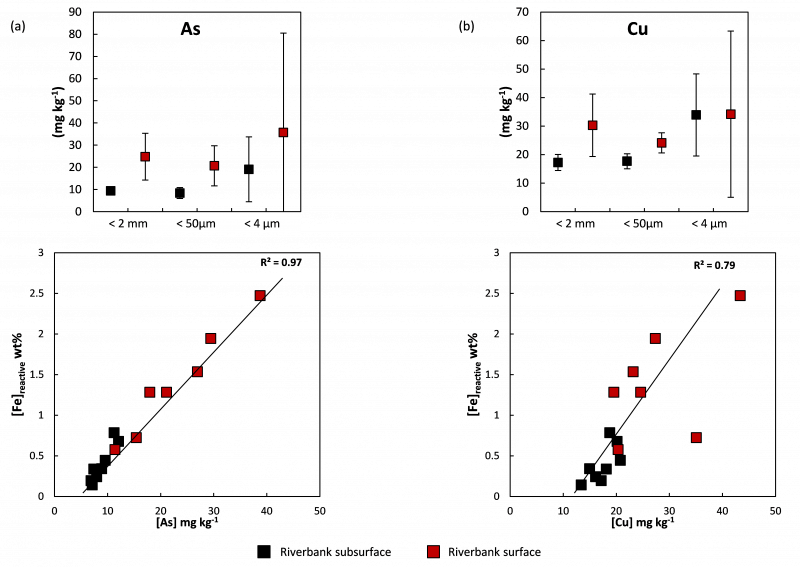
Figure 3: Content of arsenic (a) and copper (b) in riverbank samples (two particle sizes) and correlation between As (a) and Cu (b) content and the reactive Fe content.
Conclusions
Ferric deposits consist mainly of poorly crystallized structures associated with organic matter, formed rapidly on the riverbank surface and consequently acting as a biogeochemical filter. The remobilization of these Fe (III) oxyhydroxides then occurs during i) the rise in the water level and ii) erosion of the riverbanks by the river flow. The surface waters of the river are under continuous oxic conditions with a pH ranging from 6.6 to 7.6. These conditions do not promote the dissolution of Fe (III) oxyhydroxides. They are found in particulate form in the water column and can be transported to the river outlet or can sediment. In the latter case, the conditions would become anoxic and would allow the dissolution of Fe (III) oxyhydroxides and the release of the associated MTE.
As the processes involved in transporting MTE are identified, these results will be taken into account in the case of contamination of this type of sites. They will help to choose the most suitable method among the multiple existing depollution techniques (phytoremediation, biochar, nanoparticles ...).