What do the first few nanometers of the interface between a liquid and the surrounding gas look like, at the molecular level? Such a question is far from simple to be answered, and experimental techniques to obtain a “profile” of the density of the various species as a function of distance to interface are scarce.
A collaborative investigation of scientists from the Fritz Haber Institute (Berlin) shows a new method to address this problem, based on the measurement of the emission angle of photoelectrons in a liquid microjet, irradiated by soft X-rays from the PLEIADES beamline. Differences of average depth as small as 1 Å (10-10m) between different groups of atoms can be probed.
The liquid-gas interface plays an important role in many domains, for instance in atmospheric chemistry where numerous physico-chemical processes happen at the interface between liquid aerosols or the ocean and the atmosphere. Characterizing the interface, i.e. the few nanometers at the boundary of the two media, at the molecular scale remains difficult. Important contributions have been made in the last two decades thanks to the development of electron spectroscopy on liquids. Classical photoelectron spectroscopy (XPS) is a surface-sensitive technique that allows to determine which atomic species are present, and even more importantly inform on their chemical environment. This is achieved by analyzing the kinetic energy of photoelectrons ejected from atoms by X-ray irradiation. However, the information is integrated over a certain probing depth, and thus accessing the detail of how each species is partitioned to the interface is not an easy task.
A variation of XPS has been used here where additionally the angle of emission of the photoelectrons is measured. In the liquid, electrons ejected from the atoms do not necessarily follow a linear trajectory: they can be deviated by elastic collisions with surrounding molecules. This average deviation can be quantified by comparing the overall angular distribution of the electrons for the isolated molecule in the gas phase (where all electrons follow linear trajectories), and the same molecule in the liquid. The deeper an atom is located inside the liquid, the more electrons emitted from it will collide elastically before leaving the liquid. The “amount” of deviation of the angular distribution then becomes an indicator of the average depth of the probed atom. The principle is sketched in fig. 1. The layered structure (unrealistic but illustrative) of the species sketched in that figure would be difficult to derive from regular XPS measurements, but should immediately be evident in measurements of the angular distribution.
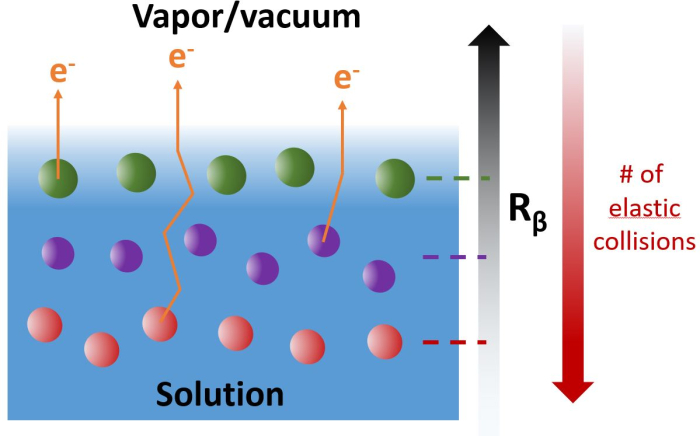
Figure 1: Sketch of the principle of the technique. Electrons coming from red atoms which are deeper in solution are more deviated than electrons coming from green atoms near the surface. This deviation caused by elastic collisions can be quantified by a factor Rβ.
What the team has shown experimentally is the validity of this principle, using a model surfactant molecule, i.e. a molecule that tends to place itself at the interface between liquid and gas, with a hydrophobic part outside and a hydrophilic part inside the liquid (see insert of fig. 2). Four out of the five carbon atoms of the molecule can be spectroscopically distinguished by XPS (as they have sufficiently different chemical environments). These four carbon groups are distributed along the interface at different depths, as is shown by detailed numerical simulations by theoreticians in the team. As shown in fig. 2, each atom exhibits a distinct degree of deviation between the gas and liquid angular distributions (quantified by the factor Rβ, which is not detailed here), even though they are only separated by a distance of about 1.5 Å.
These measurements demonstrate the potential of this technique to determine depth profiles across liquid-vapor (and other amorphous) interfaces with a sensitivity of 1 Å, i.e. one ten-billionth of a meter.
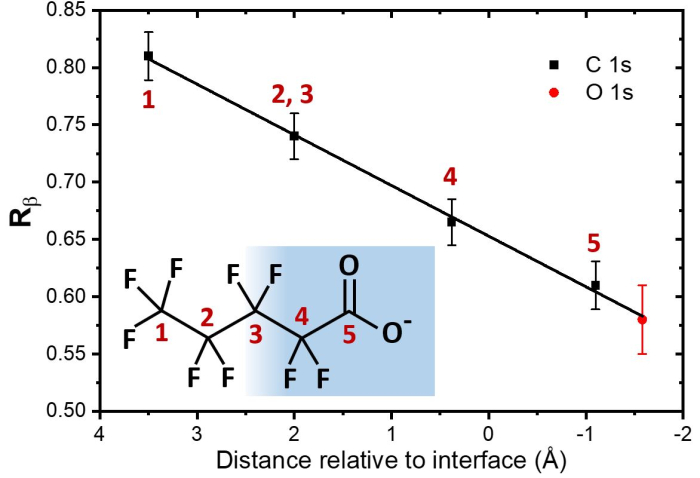
Figure 2: The Rβ factor has been experimentally measured for the different carbon and oxygen atoms of the molecule sketched in the insert (carbons 2 and 3 and the two oxygens are respectively indistinguishable and are thus grouped together). The Rβ factor varies linearly with the distance relative to the interface of the different atom groups, confirming the validity of the principle sketched in fig. 1.