Carbon dioxide (CO2) is a ubiquitous element in the Earth's volcanic systems. A large amount of volcanic CO2 is propelled from the mantle to the surface of the Earth by unique rocks called kimberlites, whose magmas can rise at the speed of sound from within the Earth and are also renowned for transporting diamonds…
Researchers from the Laboratoire de Planétologie et Géodynamique de Nantes and the Institut des Sciences de la Terre d'Orléans studied the atomic structure of these kimberlites on the LUCIA beamline, the objective was a better understanding of CO2 behaviour in these rocks and, accordingly, their role in the Carbon geochemical cycle.
The Carbon (C) geochemical cycle, i.e. the transfer of C from the Earth's interior to the Earth's atmosphere, is subject to various constraints, related in particular to the pressure, temperature and chemical composition of the environments in which C is found. CO2 behaviour in deep magmatic systems contributes to these constraints. While this behaviour is relatively well understood in kimberlite magmatic systems from a macroscopic point of view (i.e. solubility), on a microscopic scale however, there are several unexplored areas, such as:
1) the determination of the dissolution mechanisms of CO2 (present as CO32- ions), as well as:
2) the near atomic environment (i.e. alkaline and/or alkaline-earth) of the CO32- groups for these kimberlite compositions, which are very low in silica and rich in CO2.
In a series of kimberlitic glasses synthesized under high pressure conditions (up to 4 GPa, i.e. 120 km depth) and exhibiting CO2 contents ranging up to 17 wt%, the researchers studied the Mg and Ca atomic environments using the XANES and EXAFS techniques on the LUCIA beamline, in order to determine if the CO2 had a more pronounced affinity for one or other of these elements.
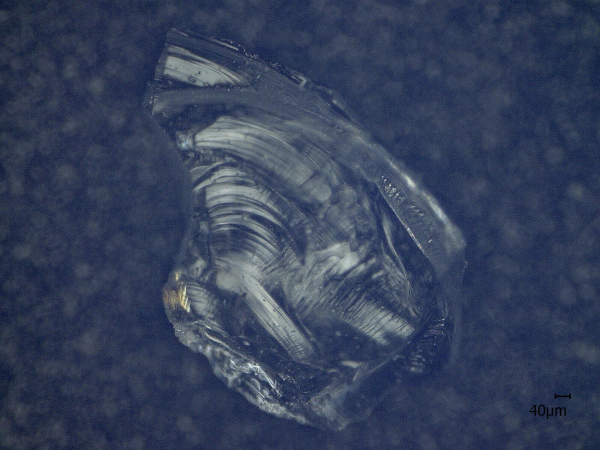
Figure 1: sample of kimberlitic glass
This study (spectra acquired at the Mg and Ca K-edges, Fig. 2), conducted with LUCIA scientists, determined that the Mg environment is not affected by the presence of CO2 whereas the Ca environment is significantly modified in the presence of CO2 (Fig 3A). Specifically, the EXAFS study of Ca demonstrates that Ca coordinates increase with the increase in CO2 (Fig. 3B).
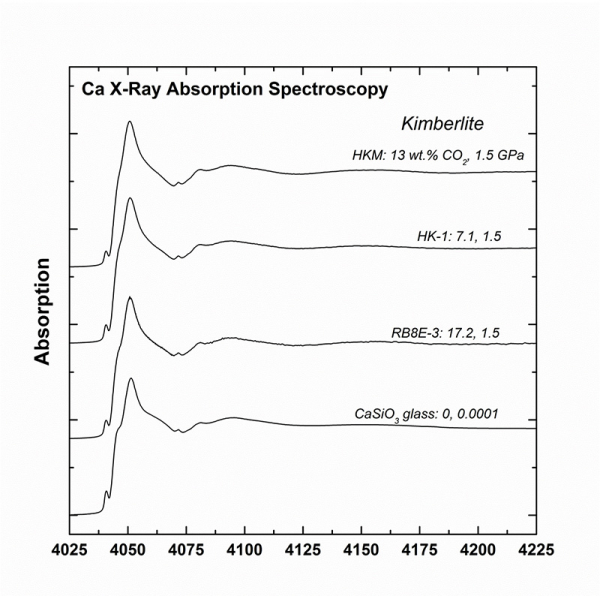
Figure 2: X-ray absorption spectra obtained on the LUCIA beamline at the Ca K-edge (~4030 eV) in kimberlitic composite glasses synthesized under high pressure conditions (1.5 GPa) and with different CO2 contents ranging from 7 to 17 wt%. The spectra acquired for a CaSiO3 glass serves as a standard and contains no CO2.
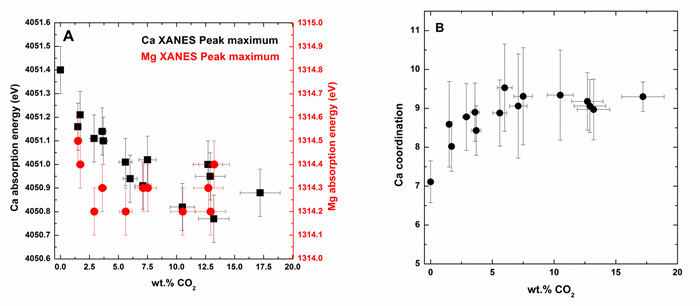
Figure 3: A) Variation in the energy position of the white line (see Fig. 1) in the Ca and Mg spectra based on the glasses CO2 content. While the position of the line for Mg remains relatively constant, the Ca line is significantly affected by the CO2 presence. B) Change in the Ca coordination number in the vitreous structure according to the CO2 content. The addition of CO2 results in an increase in the Ca coordination number.
Conclusion
The main conclusion of this study is that CO2 dissolves preferentially in the vicinity of the Ca environment within the atomic structure of kimberlite glasses. The CO2 differential affinity for Ca and Mg is mainly explained for geometrical reasons; the Ca atom environment is less dense than the Mg (bond with nearest neighbour >2.5 Å for Ca and 2Å for Mg). This lack of space in Mg is due to the very nature of the element itself, which has a much higher cationic charge per volume leading to bonds with the nearest shorter neighbours that repulse CO32- groups.
Globally, these results have an impact on the understanding of CO2 transport mechanisms in the geochemical carbon cycle on a terrestrial scale:
1) primitive terrestrial Mg-rich magma (komatiites) would have been very poor CO2 transporters in the Earth's primitive atmosphere;
2) conversely, the Ca-rich magmas of the East African rift transport a large quantity of CO2;
3) kimberlitic magmas that undergo progressive enrichment in Mg during their ascent, have their CO2 transport capacity reduced, thereby generating a fluid phase that is responsible for the explosive nature of the kimberlitic magmas.