The absence of electrical resistance in superconducting materials gives them an enormous interest in terms of applications, in the fields of energy and transport, for example. Until now, however, the known materials exhibit their superconducting properties only at extremely low temperatures (below -135 ° C.), which are incompatible with the implementation of these potential applications. Recently discovered, the hydride H3S is superconducting at -70 ° C. The groups of Pr. Timusk (Hamilton, Canada), Pr. Eremets (Max Planck Institute, Germany) and AILES beamline studied this compound using infrared spectroscopy, in order to understand the origin of this high temperature superconductivity.
Their results are published in Nature Physics
In material sciences, particular attention is given to superconducting compounds, which are characterized by two main effects: the expulsion of a magnetic field (Meissner effect) and the absence of electrical resistance. Regarding this last characteristic, applications are numerous, most notably for energy and electricity, and the potential repercussions are colossal. However, superconductivity is often induced at low or even very low temperatures, which deeply limits the conditions for its exploitation.
The quest for higher temperature superconductors has been rapidly focused towards metallic hydrogen, for which the transition is expected to be at room temperature. This study got stuck because of technological barriers, instead leading to hydrogenated compounds, as hydrides.
H3S is a promising superconductor
It is in that context that the discovery of the H3S phase, superconducting at a record temperature of 200 Kelvins, has been made in 2015 by the group of Pr. Eremets, from the Max Planck Institute for Chemistry. This team showed that when compressing hydrogen sulfur H2S up to 150 GigaPascals (more than 6 order of magnitude higher than ambient pressure) within a diamond anvils cell, a new metallic phase is formed. X-rays diffraction revealed that this structure is H3S, a hydride, exhibiting a superconducting transition below 200 Kelvins (-70°C). However, a careful characterization of this compound and in particular the determination of the energy scale of involved charges remained to be done to understand the mechanism at the origin of this high temperature superconductivity.
In that purpose, three groups (Pr. Timusk’s group, Hamilton, Canada, Pr. Eremets’ group and the team of AILES beamline) have collaborated to realize an optical study of this compound by infrared spectroscopy. In view of the complexity of the measurement, several problems had to be overcome. Firstly, the experimental setup used had to combine a control of the temperature just as the one of the pressure. Moreover, because of the use of a high pressure cell (Fig. 1a-b), the formed metallic sample is of the order of 50 micrometers. It implies that the spectroscopic study in reflectivity had to be made on a sample with a micrometric size (Fig. 1c-d). Finally, the expected spectral signatures being of the order of 3% of measured intensity, the system had to present a sufficient stability of measurement.
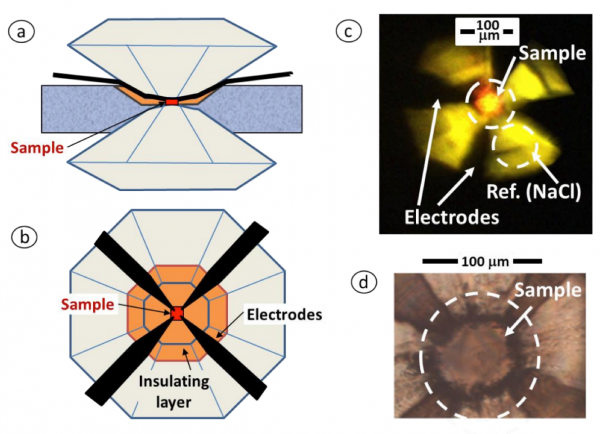
Figure 1: (a)-(b) Schematic view of a diamond anvils cell. The sample, in red, is placed between the two anvils. An insulating layer, in orange, ensures the homogeneous distribution of the pressure and electrically isolates the four electrodes, in black, which allow to measure the electrical resistivity and therefore to determine the transition temperature. (c) View by camera of the beam on the cell during the optical alignments. The synchrotron beam, in red, is reflected on the sample which has dimensions inferior to 100 micrometers. The light of an exterior source, in yellow, allows to visualize the cell during this alignment. (d) View of the same cell containing the sample under an optical microscope.
IR reflectivity spectroscopy, under high pressure
Concerning the optical study, three types of signatures have been observed. The first corresponds to the measure of the superconducting gap (Fig. 2a), i.e. the energy difference between the superconducting state and the metallic state. In the framework of this study, the energy of this gap has been determined at 73 meV, confirming at the same time the validity of the conventional theory of superconductivity for this compound. This energy, located in the far infrared, is a unique case, indeed the other superconducting gaps were observed until now in the less energetic domain of THz.
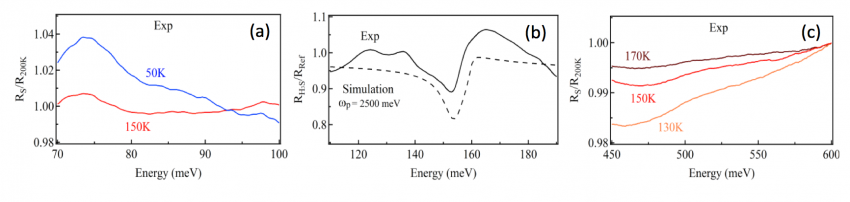
Figure 2: (a) Ratio between the superconducting and the normal states reflectivity of H3S in the far infrared range where the superconducting gap appears. (b) Ratio between reflectivity of H3S and a reference in the mid infrared. Phonons observed in the experimental curve, solid line, are in agreement with the simulation calculated for a plasma frequency of 2500 meV. (c) Ratio between the superconducting and the normal states reflectivity of H3S in the near infrared, where reflectivity decreases when temperature decreases, on the contrary to metals.
The second signature assimilated to H3S phonons (Fig. 2b), i.e. to its vibration modes in phase, is observed in the mid infrared (~150 meV). Even though it does not provide direct information on superconductivity, these phonons inform on the structure of this material. For H3S, it has been seen that their response is particularly intense, suggesting that the electrons bring an abnormally high contribution to the vibration.
The last signature of superconductivity (Fig. 2c), i.e. a bosonic excitation, is constituted by a wide structure appearing in the spectral range of the near infrared. In this domain, the reflectivity of a metal increases when it is cooled down; on the contrary, if it is a superconductor, the reflectivity decreases. That’s precisely what is observed for H3S, testifying once again of the superconductivity and providing for the first time the scale in energy in this state.
In spite of very complicated experimental conditions, the exploitation of the high brilliance from the synchrotron radiation, combined with a specific spectroscopic ensemble, has enabled to evidence of the high energy associated to superconductivity in hydrides. Therefore, this study has allowed a better understanding of the mechanism at the origin of this phenomenon.