Solar energy has been facing limits of efficiency and production cost, requiring the developing of new materials. An English and French team have had a closer look to zinc oxide, a promising material, and to its electric properties.
In order to reduce the cost of clean, renewable energy, scientists are working towards designing new solar energy cells that will both be cheaper to make and much more efficient than current solar cells that are usually made from silicon.
One of the important materials that are part of these new designs is zinc oxide, which will be used to collect the current generated by sunlight. Zinc oxide (ZnO) is already used in many applications- it is used in paint (it’s a white powder), adhesives, batteries, electronics, and on and on.
However, as much as it has been used, the fundamental properties of ZnO, and in particular, the reason why electric current lasts for a long time in the material (called a “persistent conductivity”), was still not understood. The role of oxygen was known to be important, but there were several conflicting theories as to how this was happening.
To resolve this, a team from the University of Manchester used the TEMPO beamline at synchrotron SOLEIL. They used a laser to simulate sunlight and the X-rays from SOLEIL to measure the persistent conductivity. The TEMPO beamline allowed them to change the timing between the laser and the X-rays so that they could trace how the properties of zinc oxide changed over time increments less than a millionth of a second. Because of the highly-controlled nature of the experiments at TEMPO, including the use of ultra-high-vacuum where the atmosphere around the ZnO was almost as empty as space, it enabled us to prove that it was the amount of oxygen atoms (and the lack of oxygen atoms!) within the ZnO itself that created the persistent conductivity.
The amount of oxygen atoms in the ZnO is easily controlled during the preparation of the material, which involves heating to very high temperatures (see Figure 1) and heating the ZnO in an oxygen atmosphere. Scientists changed the time of the heating in the oxygen atmosphere from 20 minutes to just 10 minutes and saw that the persistent conductivity lasted much longer. The fact that they now know how this process controls how effective ZnO is at transmitting current means that it can be prepared to be a perfect fit for new solar energy cells [1].
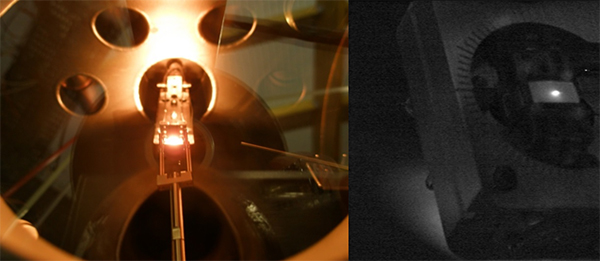
Figure 1. Left: Preparation of a piece of zinc oxide on the TEMPO beamline. Right: Zinc oxide crystal (5 x 10 mm) in the analysis chamber illuminated with laser and X-ray light.
The sunlight-absorbing component of next-generation solar cells could be so-called “quantum dots” which are tiny particles of semiconductors (with sizes less than a billionth of a metre). Quantum effects allow for different parts of the solar light spectrum to be absorbed simply by changing the size of the dots, and they are relatively cheap to make. They can also be designed to have increased efficiency, again due to quantum physics effects.
A next-generation solar cell would involve having these quantum dots (QDs) chemically linked to ZnO which is then used to transmit the electric current created in the dots. At TEMPO we attached two QD samples, one made of lead sulphide (PbS), and one made from cadmium sulfide and zinc selenide (CdS/ZnSe). In both cases researchers saw that the onset of the conductivity became much faster when the quantum dots were attached to ZnO surface. This indicates that the electric current created in the QDs by the laser (that simulates sunlight) was directly injected into the ZnO, which is exactly what the system was designed to do (see Figure 2) [2].
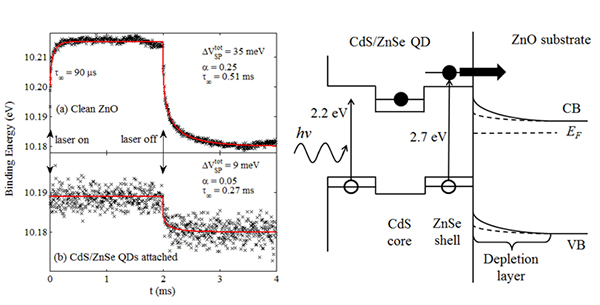
Figure 2. Left: As the laser is turned on (denoted by arrows) the binding energy of atomic core electrons shifts (a surface photovoltage, SPV), and once the laser is turned off this SPV decays on relatively long timescales (a persistent conductivity). (a) shows the clean ZnO substrate and (b) with CdS/ZnSe core/shell QDs chemically linked. The total surface photovoltage (), carrier lifetime () and material parameter () all decrease once quantum dots are attached. The timescales involved for the onset of the SPV at t = 0 ms (when the laser is turned on) becomes faster than the experimental time resolution when the QDs are attached, appearing as a step function. This indicates direct injection of electrons from the shell of the QD into the conduction band (CB) of the ZnO substrate (as shown by the energy level line-up diagram in the right panel). Right: Energy level line-up diagram for CdS/ZnSe QDs attached to ZnO. Electrons are denoted by filled circles and holes by open circles. The laser energy used (hv) was 3.33 eV. The SPV shift in binding energy is caused by a reduction in the band bending (here shown with the CB minimum and valence band (VB) maximum) across the depletion layer upon illumination (dotted lines). denotes the Fermi level which is pinned by surface states in equilibrium [2].
Both studies at the TEMPO beamline at SOLEIL have shown how QDs (which are cheap to make and can be adjusted to best absorb sunlight) attached to ZnO directly inject current when they absorb light. We have also discovered exactly how ZnO can be prepared to best transmit current, by understanding the cause of its persistent conductivity. Therefore we’ve been able to demonstrate that QDs attached to ZnO could be an ideal model for next-generation solar energy cells.