Peroxy radicals (RO2) are key reaction intermediates in the low temperature oxidation of organic compounds and play essential roles in combustion processes and in atmospheric chemistry. In the atmosphere, their reactivity can lead to the formation of a series of secondary pollutants such as ozone and secondary organic aerosols, with a high impact on the atmospheric chemical content and human health. The reaction mechanisms involving peroxy radicals are closely linked to their specific structures, which become progressively complex with increasing mass, and usually contain several structural isomers (molecules with the same raw formula but differing by the chemical bonds between the constituting atoms), plus their rotamers (molecules with atomic arrangements differing by a rotation around a given chemical bond). But, due to their complexity and in particular the instability of their cations, probing the isomers of peroxy radicals is still a real challenge, limiting our understanding of chemical reactions networks in which they might be involved.
Very recently, the above-described challenge of identifying isomers of peroxy radicals in the gas phase has been successfully tackled at SOLEIL by an international consortium of researchers from Hefei Institutes of Physical Science (Chinese Academy of Sciences), Université Gustave Eiffel, CNRS-Bordeaux, CNRS-Lille and the DESIRS beamline group. Their work has been published as a cover article in Chemical Communication.
The authors reported on the identification of the isomers of propylperoxy radical, 1-C3H7O2 and 2-C3H7O2, by using synchrotron radiation-based double imaging photoelectron photoion coincidence (i2PEPICO) spectroscopy, complemented by accurate theoretical computations. The state-of-the-art method of i2PEPICO analyzes in coincidence photoelectrons and photoions formed by each single photoionization event and has the ability to identify and decipher very dilute and elusive species in a complex chemical environment (like a chemical reactor), by providing spectral fingerprints (photoelectron spectrum - PES) of each mass-tagged constituent. This is especially useful to separate and identify different structural isomers and even rotamers, which cannot be separated by pure mass spectrometry-based techniques.
Briefly, a microwave discharge fast flow tube was employed to model chemical reactions of the atmosphere, in which the propylperoxy radical was generated through the reaction of the propyl radical with oxygen, initiated with fluorine atoms in a C3H8/O2/He mixture. Two kinds of C3H7+ cations (m/z = 43), originating from direct photoionization of the propyl radical (C3H7) and from dissociative photoionization of the propylperoxy radical (C3H7O2) whose cation is not stable, have been observed in the experiments and can clearly be separated in the photoionization time-of-flight (TOF) mass spectra and ion images through their different ion kinetic energies, as seen in Figure 1.
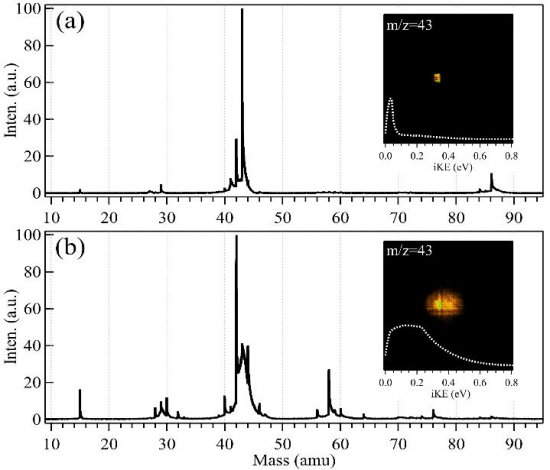
Figure 1: Synchrotron photoionization TOF mass spectra and the corresponding m/z = 43 ion images acquired (a) without and (b) with addition of oxygen into the flow tube.
The mass-selected threshold photoelectron spectrum (TPES) corresponding only to the propylperoxy radical was then measured in coincidence by scanning the photon energy as shown in Figure 2. A vibrational fine structure can clearly be observed in the 9–11 eV energy range of the TPES benefiting of the high energy resolution of the DESIRS beamline and of the i2PEPICO setup, called DELICIOUS 3, available on this beamline.
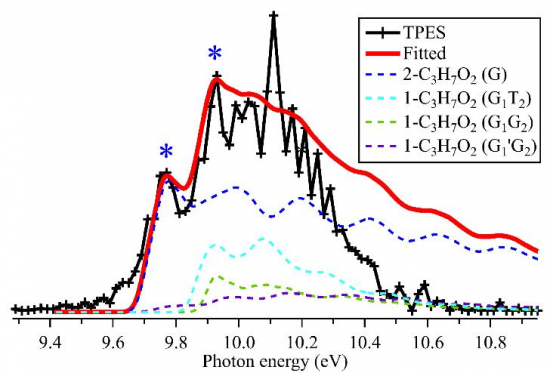
Figure 2: Threshold photoelectron spectrum of C3H7O2 radical and its fitted calculated contribution of the various isomers/rotamers.
In order to identify the detailed structures of the propylperoxy radical, high-level theoretical calculations were performed and predicted that, in the flow tube, the propylperoxy radical should be produced as two isomers, 1-C3H7O2 and 2-C3H7O2, together with their individual rotamers, i.e. the G1G2, G1T2, T1G2, T1T2 and G1’G2 of 1-C3H7O2, and the G and T of 2-C3H7O2, as shown in Figure 3. The stable structure of these rotamers and their corresponding cations, their individual energetic levels and potential energy curves involved in their photoionization, have also been theoretically calculated and discussed.
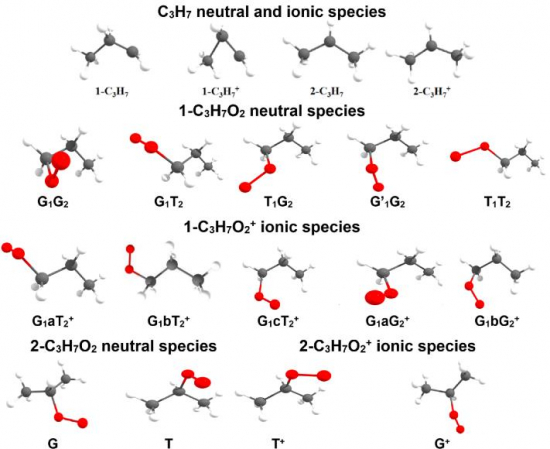
Figure 3: Calculated stable structures of the isomers of the propylperoxy radical, together with their cations.
In addition, the Franck-Condon factors* for the ionization transitions of the two isomers 1-C3H7O2 and 2-C3H7O2, from their neutral X2A’’ ground state to the cationic a1Aʹ excited state (the only one to be efficiently populated via vertical transitions), have been calculated. They indicate that only the G1G2, G1T2, G1’G2 (for the 1-C3H7O2 isomer) and G (for the 2-C3H7O2) rotamers show favourable Franck-Condon factors upon photoionization. The calculated photoelectron spectra of these rotamers, obtained from their Franck-Condon factors, are presented in Figure 2 and show a very satisfactory agreement with the experimental global TPES, confirming the identification of the isomeric distribution of the propylperoxy radical present in the reactor. Moreover, the comparison between the fitted and experimental spectra confirm that the first two peaks of the TPES correspond to the a1Aʹ state of 2-C3H7O2+ (Adiabatic Ionization Energy (AIE) = 9.759 ± 0.005 eV) and that of 1-C3H7O2+ (AIE = 9.919 ± 0.005 eV)
The present study exemplifies the capabilities of i2PEPICO complemented by advanced computations, as a powerful method able to provide new insights into on-line analysis of complex gas-phase chemical reactions, such as combustion processes and atmospheric oxidation, through isomer/rotamer identification of elusive radical intermediates.
* The vibrational wavefunction overlap between the neutral and the cation for a given electronic transition. The Franck-Condon factor gives the probability to populate a given vibrational state of the cation.
This work was supported by the National Natural Science Foundation of China (No. 21773249, 91961123, 91644109), the International Partnership Program of Chinese Academy of Sciences (No. 116134KYSB20170048) and the French Agence Nationale de la Recherche (ANR) under Grant No. ANR-12- BS08-0020-02 (project SYNCHROKIN).