To build the hard scaly shells that enclose their cells, coccolithophorid algae produce calcitic scales within an intracellular calcifying compartment and secrete them onto the cell surface. The formation of the scales requires the movement of large amounts of calcium from the surrounding seawater into the calcifying compartment. The pathways supplying this compartment with calcium remain elusive.
A team of researchers from the Max Planck Institute of Molecular Plant Physiology (Germany), the Max Planck Institute of Colloids and Interfaces (Germany), the German Research Centre for Geosciences (Germany), and the beamline LUCIA have found first evidence that a recently discovered compartment, containing a calcium-phosphate (Ca-P rich) phase, participates in the calcium supply to the calcifying compartment. This work has been published in the journal Advanced Science.
Coccolithophores are single-celled algae that inhabit the sunlit layers of the oceans worldwide. The hallmark of these algae is an extracellular shell from a collection of little mineralized disks known as coccoliths (Fig. 1A). Each coccolith is an array of nanoscopic crystals of the mineral calcite and is produced inside the cell within a specialized compartment called the coccolith vesicle. Most aspects of coccolith formation are still elusive including the pathways along which the constituent ions move before ending in the coccolith calcite.
The biologically controlled formation of calcite is a widespread phenomenon and best investigated for multicellular organisms. Recent studies on the biomineralization mechanisms in various multicellular calcifiers have revealed a widespread use of amorphous precursor phases as intermediates in the formation of crystalline biomaterials. Very recently, the team of researchers discovered a compartment in the coccolithophore Emiliania huxleyi, which is the most abundant calcifying organism on Earth, storing an amorphous Ca-P-rich phase. This discovery prompted the question of whether or not the Ca-P pool is a source of calcium for coccolith formation.
To address the question of material transfer between the Ca-P-rich compartment and the coccolith vesicle, the researchers conducted pulse-chase experiments with Sr (Fig. 1B) and used cryo X-ray absorption spectroscopy (XANES) and analytical transmission electron microscopy to follow the Sr within the E. huxleyi cells. Sr ions served as a marker for Ca because they are transported by Ca channels and can be transferred into the coccolith vesicle as is evidenced by the incorporation of Sr in coccolith calcite.
The investigation of thin sections of E. huxleyi using analytical electron microscopy showed that Sr accumulates in coccolith calcite and also in the Ca-P-rich compartment, but not in other intracellular locations. This showed that the Ca-P-rich compartment accommodates the majority of intracellular Sr outside the coccolith vesicle. To determine the chemical environments of the cellular Sr at different time points in the pulse chase experiments cryogenic strontium L2,3-edge XANES was used (Fig. 1C). In parallel with the Sr pulse, coccolith formation was switched from “off” to “on”. The results of the pulse-chase experiments showed that the environment of Sr in the non-calcifying (coccolith formation is turned off”) cells is highly similar to Sr in an amorphous phase. As calcification commences in the chase period, the Sr signal becomes similar to Sr incorporated into calcite (Fig. 1D,E). Having found that Sr ions are initially present in the amorphous environment of the Ca-P-rich phase and move to a crystalline environment with progression of calcification, the conclusions from our experiments are that Ca is transferred from the Ca-rich pool into the coccolith vesicle and that the Ca-P-rich compartment is a component of the coccolith biogenesis pathway.
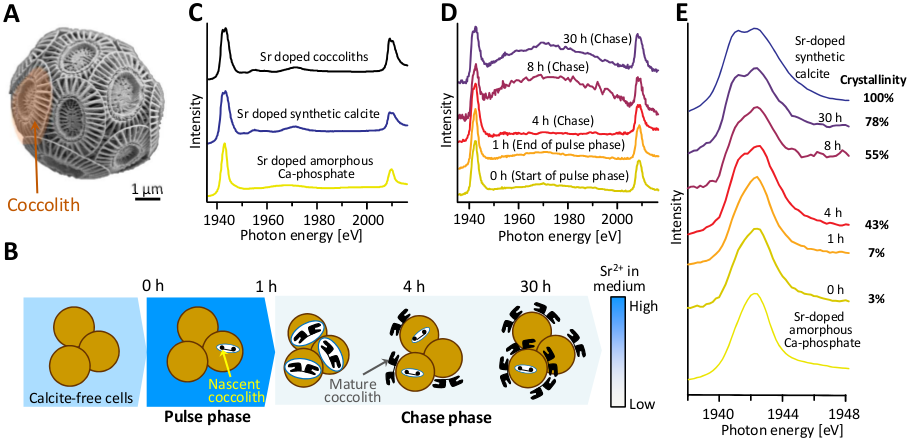
Figure 1:
A) Scanning electron micrograph of the coccolith shell surrounding the alga Emiliania huxleyi.
B) Design of the pulse chase experiment with Sr. Cells were grown at conditions that arrest coccolith formation and yield coccolith(calcite)-free cells. Calcification and the Sr pulse were induced simultaneously through the addition of CaCl2 and SrCl2 (0 h). After 1 h the Sr2+ concentration in the medium was lowered below the detection limit. The Ca2+ concentration was kept constant and therefore cells proceeded with calcification.
C) Sr L2,3-edge XANES spectra of frozen reference samples and D) cells from different time points of the pulse chase experiment. E) Detailed scan of the L3-edge of the samples as in (D).
These findings not only provide fundamental insights into the mechanism of coccolith formation but also have implications for fields beyond biomineralization.
This novel perspective on the supply of the coccolith vesicle with material is indeed of relevance for the interpretation of the trace metal content of fossil coccoliths in the context of paleoclimate reconstructions and for understanding and predicting the response of coccolithophores to global environmental changes such as ocean acidification.