X photoemission spectroscopy experiments carried out on TEMPO beamline provide the first direct and measurable evidence of an existing link between the organic and mineral phases of calcareous biominerals, such as corals or mollusk shells. These results are published in Analytical and Bioanalytical Chemistry.
Many living organisms (mostly marine) are able to create mineralized structures dedicated to specific biological functions as illustrated by the protective shells of mollusks (1a, b, c) or the calcareous substrates built by corals (1d, e) whose mineralizing activity results in massive reefs of great geological importance (1f). Bones and teeth also exemplify these biomineralization processes which obviously imply a close control exerted on deposition of the mineral phases, allowing the mineralized organs to fulfill their functions.
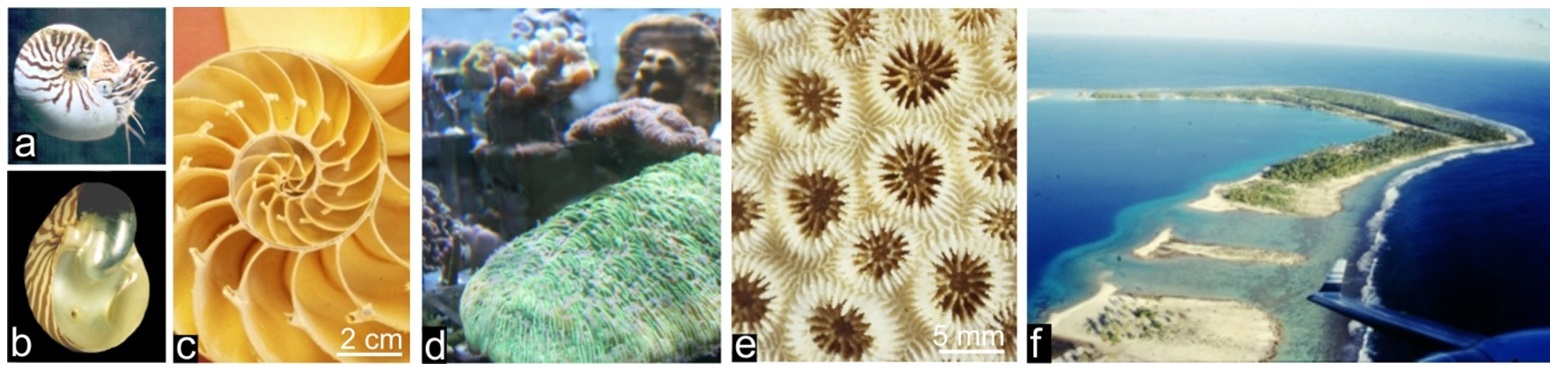
Figure 1
Nautile (a) and its shell (b-c); Corals (d-e) and corallian atoll in Polynesia (f). Note that such geologically important formations (e.g. the several thousand kilometer long Australian Great Barrier reef) are entirely built by biologically produced carbonates.
From morphological diversity to common microstructural features
We are all familiar with the species-specific morphologies of calcareous shells, but what is surprising is that, when observed with a microscope, these calcareous hard-parts are not simple aggregates of Ca-carbonate particles. They appear to be built by crystals whose well defined sizes, shapes and three-dimensional arrangements are also species-specific (Fig. 2). In spite of a century long series of investigations, the mechanism of this biological control on crystallization is largely enigmatic yet.
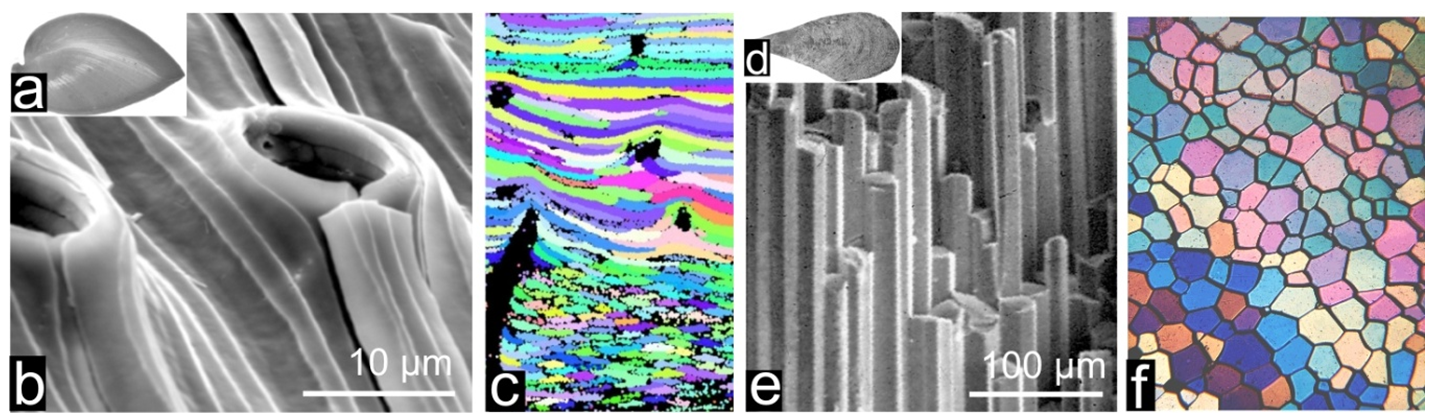
Figure 2
In a Brachiopod shell (a) calcite fibers (b) exhibit a monocrystalline pattern (c: polished surface, back-scattered electron diffraction) in spite of a sinuous growth. In the Mediterranean shell Pinna (d) calcite is deposited as strong linear prisms (e) with a monocrystalline behavior (f: transversal thin section, transmitted polarized light). Species-specific morphologies of the shell building units make obvious the biological control on Ca-carbonate crystallization.
In addition to specific morphologies and spatial arrangements, calcareous biocrystals do not follow the usual growth mode of crystals as it occurs in saturated solutions. Here (e.g. in the coral skeleton Fig. 3a-b) length of the crystalline units (3c) is simultaneously increased by common growth layers (3d). Extended investigations have shown that calcareous skeletons always comprises an organic component mostly made of proteins and sugars associated in different proportions depending on species, with recently evidenced lipids. Remarkably, synchrotron-based X-ray absorption mapping allow to establish that organic compounds (here sulfated polysaccharides: 3f) are distributed in exact correspondence with the mineral growth layer (3g). Clearly, interplay between mineral and organic component (admittedly the leading factor in the biological control of crystallization) occurs at an infra-micrometer length-scale.
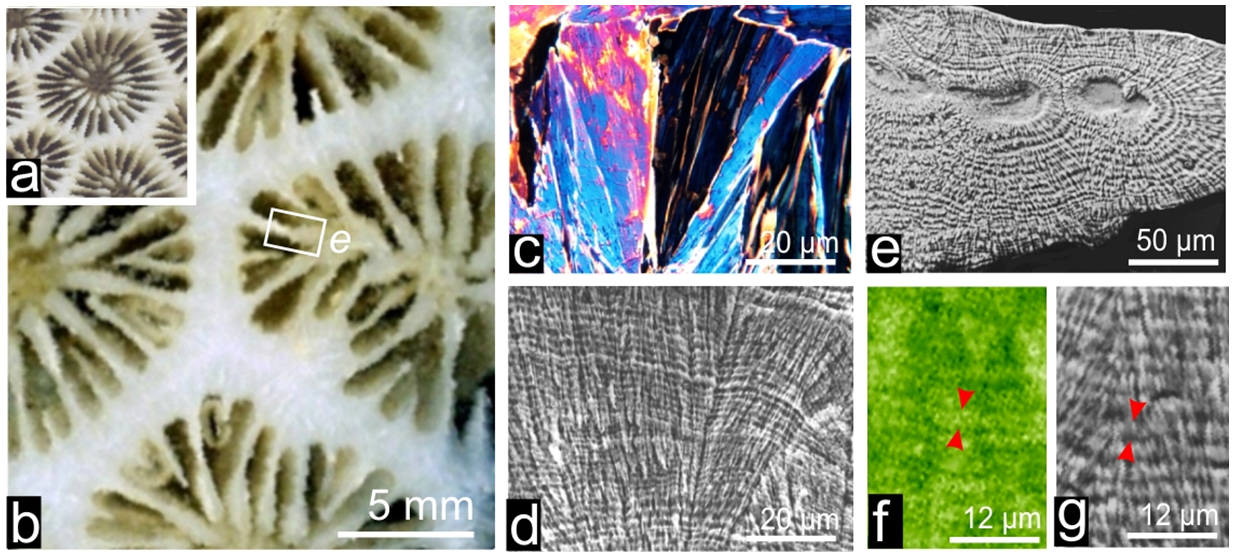
Applying atomic force microscopy (AFM) to biogenic carbonates produced by any species -here illustrated by Pinna prisms (fig.4a-b) but no exception is known so far- allows illustrating the infra-micrometric structure of these materials. In contrast to what could be expected from the crystalline appearance of these shell building units, they are built by densely packed nodular grains with no external feature revealing their crystalline nature (e.g. angular faceting). AFM phase contrast imaging also establishes the presence of a tip-interacting component, viewed as grey to black coating of the grains (fig.4c).
In agreement with current concept of a transient amorphous phase predating the crystalline status of biominerals, TEM imaging and diffraction carried out at the growth front of Pinctada calcite prisms provide a realistic nano-scaled illustration of crystallization as it may occur from the passage of an amorphous mineral (fig.4d-e) to a crystallized one. Between amorphous periphery of the grains and their crystallized internal area, the yellow arrows (fig.4e) draw attention on the key process which is going on at this place: the amorphous mineral phase stabilized -up to this moment- by the organic compounds is led to leave its organic carrier and to transform into a crystalline phase (no information presently exists about the mechanism triggering this process). Formation of the crystal lattice determines a strong segregation within the micrometer thick growth layer of the skeleton: excluded from the growing crystal lattice organic components are compacted at the periphery of the crystallizing mineral phase, resulting in an organic-rich amorphous envelope surrounding the crystalline grains. The mineralizing matrices that investigators have collected during decades by decalcifying the shells is nothing other than this organic component which has ensured an essential function in the formation of the shell growth layers and remained occluded inside the shell building units (but not within the crystalline lattice).
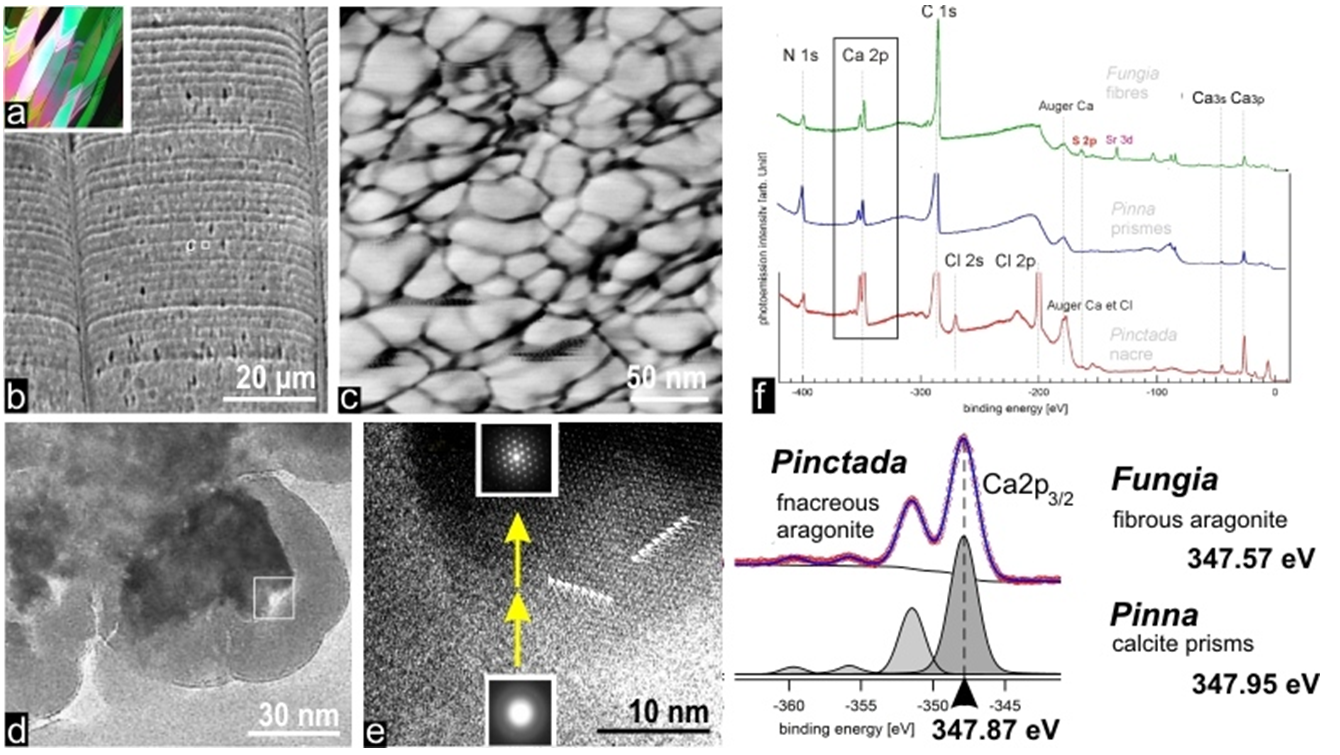
Figure 4 : Ultrastructure nodulaire des strates de croissance.
Recent XPS experiments carried out at TEMPO beamline open a first view on this key phase of the biomineralization process by providing information on the change which occurs in 2p orbitals of the calcium still attached to the organic matrix freed by decalcification, compared to Ca as free element or inserted in carbonate lattice of calcite or aragonite.
Interestingly, Ca2p binding energy is significantly modified with respect to reference values but even more important, modification of the Ca2p binding energy is taxonomy dependent, i.e. the shift value depends upon the biological origin of the matrix (Fig. 4f).
Perspectives
In this short series of pictures illustrating the top-down approach of calcareous biominerals, the TEMPO experiment reaches the ultimate resolution: the relationship between organic and mineral phases established at the molecular level. Additionally, and certainly even more important, this result is nothing other than the first direct and measurable evidence of an existing link between the two components, all previous documents establishing only their correlated distributions.
Among various areas in which calcareous biominerals are involved, their role as recorders of sea-water properties is of major importance in the present context of environmental changes. Worth to note that interpretation of chemical measurements made on biominerals largely depends upon a reliable concept of bio-crystallization. In the historical paper reporting the first application of chemical measurements made on biominerals (1951) Urey made the truly prophetic statement that “a vital effect may exist” during formation of biominerals, leading to what he named the “vital effect” influencing the crystallization process. As a result, from a practical view point, environmental geochemists dealing with biominerals are still constrained to a purely empirical practice (calibration).
The recent XPS data represent a perfect illustration of this vital effect at the molecular level. Allowing an accurate and reliable approach to molecular changes which occur during formation of calcareous biominerals the synchrotron-based XPS method may bring a significant contribution to an improved model of biocrystallization and a more efficient use of biominerals as natural archives of environmental history.
Recherche réalisée dans le cadre d'un contrat ANR (programme blanc n° 11BS1000502 - 3D- PtyCCoBio) sous la responsabilité de V. Chamard, Institut Fresnel, Marseille.