Subduction zones are extraordinarily complex shear-zone regions in the Earth. To understand their dynamics, it is important to investigate the deformation behavior and microstructure of rocks being deformed within these complex regions at high pressures and temperatures (HP-HT). To do so, researchers from the University of Lille, CNRS, Sorbonne University and SOLEIL carried out X-ray tomography experiments on serpentinized peridotite at PSICHE beamline. Their results may lead to a better understanding and constrains on subduction initiation and dynamics in the Earth lithosphere.
This work, funded by ANR, was presented by Tommaso Mandolini, PhD student at the University of Lille, at the American Geophysical Union (AGU) Fall meeting 2020. With the results presented, Tommaso Mandolini won the Outstanding Student Presentation Award (OSPA) of the Mineral and Rock Physics section of the AGU.
Two tectonic plates, one plunging underneath the another, making up for the most seismic and volcanic activities that occur in the entire planet. They carry, recycle and deform materials and minerals aggregates (i.e., rocks) through the Earth interior, and their dynamics is strictly linked to the evolution of our planet. To understand subduction zones dynamics, it is important to investigate the deformation behavior and microstructure of rocks being deformed within these complex regions at high pressures and temperatures (HP-HT). Although rocks are made of multiple minerals and can be highly, mechanically heterogeneous, in a simpler scenario just two main mineralogical phases may be involved in the deformation of a rock: one phase to be weak and the another strong, with the former to be more susceptible to flow under deformation. Then, with increasing deformation and depending on the weak phase content in the rock, the weak mineral may eventually play a dominant role in the bulk deformation behavior of the aggregate, accommodating most of the deformation and forming a microstructure of interconnected weak layers (IWL).
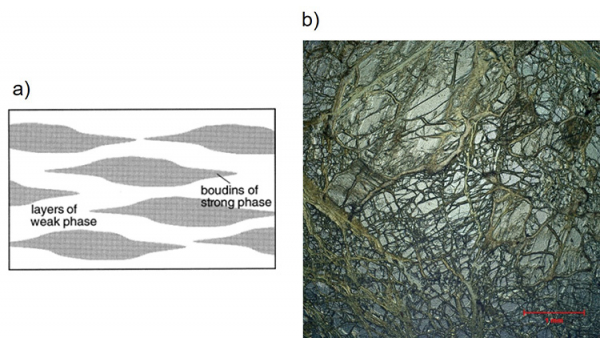
Figure 1:
(a) Simplified illustration of IWL texture showing the weak phase (white) forming interconnected weak layers among the strong phase (grey). Image modified after Handy et al. (1994).
(b) Image of a natural serpentinized peridotite showing serpentine forming a clear network of veins among the stronger olivine grains, which resembles the texture of IWL shown in (a). Image from blogs.agu.org/georneys/2012.
The IWL texture (Fig. 1a) and its onset are really important to understand subduction zones dynamics, for instance, in the lithosphere. In fact, the formation of interconnected layers in the weak mineral results in strain localization in the rock, which may lead to large-scale shear-zones that ultimately enable subduction initiation in the Earth lithosphere. One of the dominant rocks present at subduction zones is serpentinized peridotite (Fig. 1b), which is mainly made of minerals of olivine, i.e. the most common magnesium-iron silicate in the Earth mantle, and serpentine, which is a group of hydrous magnesium-iron phyllosilicates. Antigorite, the high-pressure variety of serpentine, coexists with olivine in serpentinized peridotite and is weaker than olivine under deformation occurring in nature.
With the data collected at SOLEIL on the PSICHE beamline using in-situ HP-HT X-ray tomography, the evolution of microstructure and behavior of aggregates of olivine (strong) + antigorite (weak) under torsional deformation at high strain regimes was investigated. The aim was to observe at which experimental conditions and volume content of antigorite an IWL texture may be likely achieved in the samples when deformed at conditions close to subduction zones in the lithosphere, which means pressures up to 4-5 GPa (i.e. 50,000 times higher than atmospheric pressure) and temperatures up to 600°C.
The main results suggest that the total interconnectivity (or connectivity) of the weak antigorite increases with strain in all samples investigated, with the higher interconnectivity reached at higher antigorite content (Fig. 2a). Here, the antigorite forms clearly visible interconnected weak layers where strain and deformation localize (Fig. 2b), in agreement with a IWL texture.
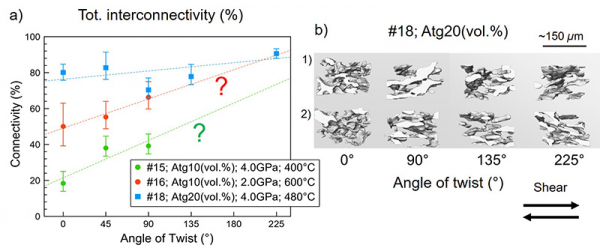
Figure 2:
(a) Total extent of interconnectivity evolution in the weak antigorite with increasing angle of twist imposed during torsional deformation. The angle of twist here is an indication of the increasing extent of strain potentially transferred to the samples. Inset showing sample numbers, with antigorite (Atg) contents, and estimated experimental conditions of pressure and temperature. Question marks indicate estimated trends until maximum angle of twist for two samples.
(b) Volume rendering of antigorite showing its morphology evolution with increasing angle of twist in two scenarios (1 and 2) as shearing deformation is applied (arrows).
To link these observations on the microstructure with the deformation mechanism of the phases, electron microscopy analysis was additionally performed at the University of Lille to get information on the misorientation crystalline lattice and plasticity of the crystals in the aggregate due to deformation. It appeared that in the sample with higher antigorite interconnectivity the stronger olivine shows localized intracrystalline deformation indicating a local low-temperature plasticity behavior.
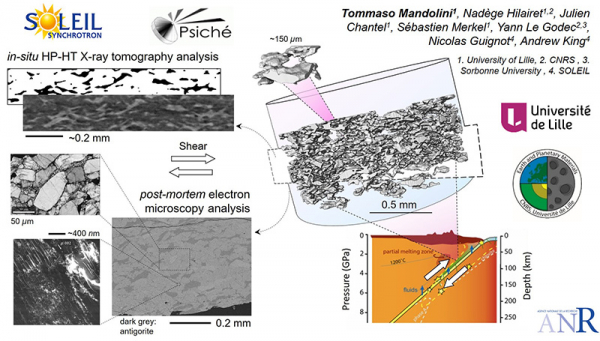
Figure 3: Overview of the multiple-scale observation methodology used in this work to analyze and investigate the samples (from millimeter down to nanometer) to better understand multi-phase rock deformation at subduction zones.
Right-hand side: bottom, representation of a subduction zone shearing-region with white arrows indicating the sense of shear deformation (image modified after Poli and Schmidt, 1998); top, volume rendering from X-ray tomography showing antigorite morphology in the all sample.
Left-hand side: top, 2-d section of X-ray tomography images showing microstructure of deformed antigorite; bottom, electron microscopy images at different scales showing areas in the sample from mm-sized down to nanometer-sized (left-bottom corner), this latter in transmission electron microscopy (TEM) mode showing dislocation microstructures in one olivine crystal.
These findings will give new insight on strain localization and deformation mechanism at multiple scales of observation in multi-phase rocks representative for subduction zones (Fig. 3), which ultimately may lead to a better understanding and constrains on subduction initiation and dynamics in the Earth lithosphere.
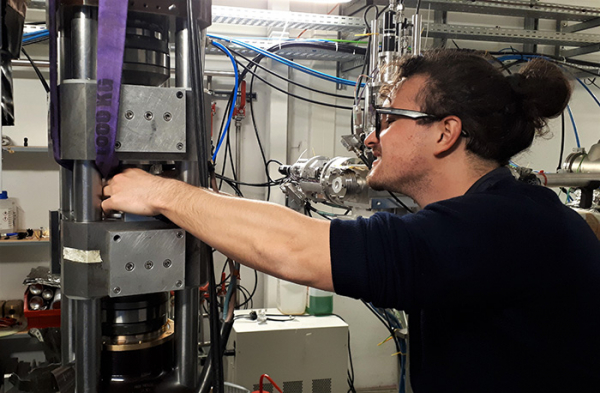
Figure 4: Tommaso Mandolini inserting a sample between the anvils gap of the torsion press apparatus (the RoToPEc) used for the high pressure experiments on PSICHE beamline.