Researchers from South Korea’s Gwangju Institute of Science and Technology (GIST), Korea Advanced Institute of Science & Technology (KAIST), and Laboratoire de Chimie Physique Matière et Rayonnement (LCPMR) at Sorbonne Université cooperated together to reveal a piece of crucial information for developing a novel catalyst. This information can dramatically increase the conversion efficiency of carbon dioxide and shows promising signs of its effectiveness. Some of their results were obtained on TEMPO beamline at SOLEIL.
The development of greenhouse gas conversion technology is one of the most important topics among OECD countries and the G7. These highly active industry-academia-research, public-private partnership researches devote important resources to achieve the carbon-neutral global standards by 2050. Among numerous approaches, the conversion of carbon dioxide (CO2) into commercially valuable products, such as ethanol or methanol, is a highly sought-after technology since it not only helps us step forward to carbon neutrality but also creates something that can be used in our daily lives.
Nonetheless, the conversion of CO2 into industrially useful chemicals poses a big challenge due to the intrinsic nature of CO2; for example, CO2 is extremely stable and cannot easily be dissociated. Also, no ideal catalyst has been pinpointed to promote the dissociation of CO2. Researchers from GIST, KAIST and Sorbonne Université undertook this matter to provide a critical information on how the catalyst should be prepared to promote the dissociation/conversion of CO2. The team utilized the ambient pressure X-ray photoelectron spectroscopy (NAP-XPS) at TEMPO beamline at SOLEIL and the ambient pressure scanning tunneling microscopy (AP-STM) in Korea.
The joint research team focused on the fact that the reaction activity of CO2 molecules can vary depending on the surface structure of the catalytic material. With the use of AP-STM, the team captured, in real time, the decomposition process of CO2 molecules reacting with the ultra-thin Cu surface, featuring steps (Figure 1) about 0.1 nanometre thick, i.e. 1/1,000,000 the thickness of a human hair (a hair is ~ 0.1 mm). Also, in order to identify the chemical conversion process at the stepped Cu surface, the team used the NAP-XPS located at TEMPO beamline at SOLEIL to identify the chemical bonds formed when the ultrafine stepped Cu surface reacts with CO2.
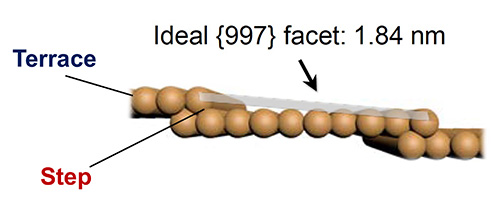
Figure 1: diagram of the catalyst surface (Cu({997)}), made up of copper atoms arranged in a succession of overlapping "terraces" to form "steps". The height of a step is less than a nanometre.
From the joint research efforts, the research team found that the dissociation of CO2 – which begins at the edges of the steps and propagates to the terraces of the surface – leads to the massive reconstruction of the surface that modifies its morphology, associated with a unique chemical signature. This behavior was demonstrated by NAP-XPS at SOLEIL, when the surface was exposed directly to CO (see figure 2), the product of CO2 reduction. CO is indeed the key element, as it generally neutralizes the surface by adsorbing onto the active sites. It is therefore essential to study the behavior of a surface exposed to CO. The team was able to show that CO molecules adsorb preferentially on the edges of the steps, but can migrate and induce a rearrangement of the copper atoms, producing new adsorption sites. The new morphology of the catalyst surface improves the yield of the CO2 reduction reaction.
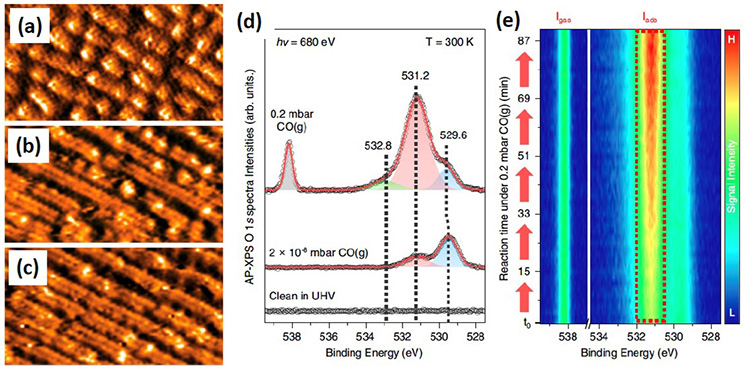
Figure 2. Time-lapse morphology observations on the Cu(997) surface under 1 mbar CO gas conditions (T = 300K).] Left: AP-STM images taken at 29 (a), 37 (b), and 66 min (c) after filling the reaction cell with CO gas. (d) Collected O 1s core-level NAP-XPS spectra (hν = 680 eV) in UHV (bottom) and under CO gas conditions of 2.10−8 (middle) and 0.2 mbar (top). The peaks correspond to the adsorption of atomic oxygen by CO dissociation (529.6 eV), CO molecules adsorbed at different sites (531.2 and 532.8 eV) and CO molecules in the gas phase (538.2 eV) (e) Time-dependent measurements of O 1s core level spectra at 0.2 mbar CO pressure.
The initial dissociative reaction pathway of CO2 observed by the team is a new discovery that goes beyond the existing understanding of the carbon dioxide catalyst phenomenon on Cu surface. Indeed, these investigative results provide convincing evidence of the influence of surface morphological structures at the atomic level, providing important insights for the development of high-efficiency CO2 catalysts.
The dissociation of CO2 on Cu nanocatalysts consisting of facets can thus open up the prospect of improving CO2 conversion yields into future sustainable energy sources in industrial processes, an improvement that will help reduce the current global warming caused by greenhouse gases.