By carefully choosing the photon energy for the excitation of the deep-core 1s electron in HCl to the dissociative σ* orbital, scientists from France (UPMC/CNRS and SOLEIL), Germany (Berlin University) and Brazil (UFABC, Sao Paulo) showed they can control the speed of the nuclei and partially either quench or stimulate H-Cl bond dissociation. The manipulation of molecular fragmentation in the tender x-ray energy domain on a sub-femtosecond time scale was demonstrated for the first time. Their results, obtained on GALAXIES beamline, are published in Physical Review Letters.
Using X-rays of about 3 keV, a Cl 1s electron in HCl molecule can be promoted to the unoccupied valence orbital, called σ*. This orbital is anti-bonding, meaning that any electron present there weakens a chemical bond and urges molecular dissociation by pushing the atoms apart. The created Cl 1s deep core-electron vacancy is highly unstable and is very rapidly filled by another electron residing on one of the shallower electronic shells of the HCl molecule. This shallower core-electron vacancy is also unstable and has to be rapidly filled. Thus, cascading of electrons is triggered through different electronic levels until no more core-electron vacancies exist in the molecule. The deeper the initial core vacancy, the more electronic levels the cascades can go through and the more intermediate unstable states can be created with different charges and even multiple core holes. The most probable chain of Cl 1s electronic decay process comprises 3 steps and lasts for about 6 to 20 fs (1 fs = 10-15 s) in HCl. At the same time H—Cl bond is elongating with increasing speed until completely broken, which may happen even before the electronic decay cascade is finished. Hence, the last steps of the cascade process are partly occurring in the already dissociated Cl-atoms, which are no longer bound to hydrogens and do not even feel their presence anymore. However, our work demonstrates that these free Cl-atoms, formed by the turbulent decay of the deep Cl 1s core-electron vacancy, carry the memory of the very first hundreds of attoseconds (100 as = 0.1 fs) of the beginning of their formation.
Controlling the ultrafast nuclear dynamics
By carefully choosing the photon energy for the excitation, one can control the speed of the nuclei and partially either quench or stimulate H—Cl bond dissociation. In particular, to match the maximum of the Cl 1→σ* transition, the energy of 2 824 eV is required. However, deviations from this energy by only 2 eV have drastic effects on nuclear dynamics. This is because different excitation photon energies promote the HCl molecular system to different parts of the core-excited state potential. If we imagine that the dissociative core-excited state potential is a downhill road, then carefully choosing the excitation energy would mean choosing a starting point for our molecule on this descending road. Slightly higher energies would place our HCl molecule higher on the hill, and lower energies – would place it lower. As the typical hill has a bell shape, the higher part of the slope is steeper compared to the lower side, where it gradually gets shallower and shallower until becoming flat. Therefore, placing the system higher on the slope would enable larger accelerations on the steeper parts of the slope leading to higher speeds within the same time frame.
For instance, using a lower photon energy (2 822 eV), results in a velocity of 1.6 km/s of the H atom after 300 attoseconds (as), i.e. 0.3 fs, when a 1s core vacancy is filled, and 16.2 km/s at the moment when the 1st 2p core vacancy is filled after about 4 fs. In comparison, 2 eV higher photon energy (2 826 eV) in respect to the maximum of the Cl 1→σ* transition results in the velocities of 2.5 km/s, and 20.5 km/s at the same time intervals of 0.3 and 4 fs, which is correspondingly 50% and 30% faster than that for the negative detuning!
Conclusions
Figuratively, this work shows that with higher energy the system would most likely take the fast lane. Contrary, if the energy is below the optimum, the system would preferably go on a slow pace. Choosing a fast lane augments the probability for fragmentation, while a slow pace increases the chances for survival of the system as whole. However, the control has only a limited range. Having a way too high or too low energy would make it more difficult for the system to embark on this narrow downhill road: with largely insufficient energy it would not be able to hit the road, while markedly excessive energies could lead the system somewhere else on another road (i.e. in relation to molecule, ionise or excite another resonant transition)
This work can be a promising start for further studies of controlled photo-fragmentation using hard x-rays.
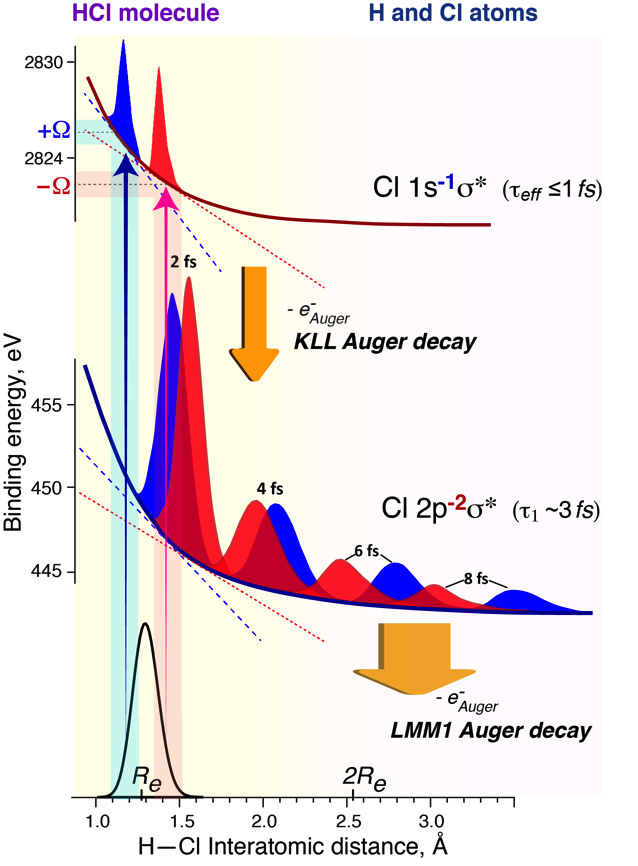
Figure 1: Diagram describing the effect of the excitation energy detuning on the wave-packet dynamics during the first two steps of the KLL Auger decay cascade path. The wave packet corresponding to the positive detuning (blue) takes over the wavepacket corresponding to the negative detuning (red) and reaches faster the bond distance of 2Re, where the molecule can be considered dissociated, despite the initial spatial distribution of the former at shorter H-Cl bond distances.
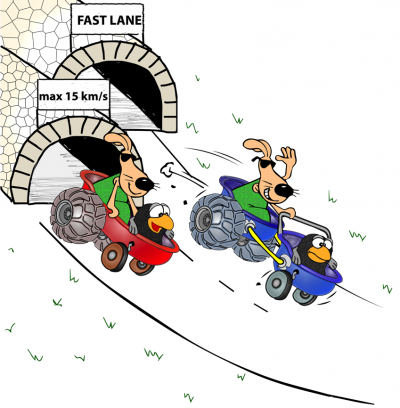
Figure 2: Cartoon, showing a figurative comparison of the wavepacket dynamics with the downhill descent of vehicles.