The effect of rising greenhouse gas emissions on global warming drives current research into improved means of separation, capture and/or storage of CO2. Porous metal-organic materials, which are made of metal nodes connected through organic linkers, are widely studied and developed for these purposes. In particular, the so-called MOPs (for metal-organic polyhedra) possess an internal cavity that gives them intrinsic porosity, while their molecular nature provides easy processability. This makes MOPs excellent candidates for developing membranes for gas separation.
Within the wider family of metal-organic cages, cuboctahedral rhodium-based MOPs (Rh-MOPs), which are assembled from 1,3-benzenedicarboxylates and dirhodium paddlewheels, offer very interesting possibilities for separation processes. Indeed, Rh-MOPs can be used for selective entrapment of gases/molecules within their cavity, while their chemical robustness enables sustained performance even when exposed to harsh conditions, such as exposure to water, acids, or competing coordinating ligands. In addition, the surface of Rh-MOPs can be functionalized to make them soluble in water or in polar/non-polar organic solvents, which increases their processability and enables using them under homogeneous conditions or shaping them into functional macroscopic objects.
A Spanish collaboration between the supramolecular nanochemistry and materials group at the Institut Català de Nanociència i Nanotecnologia (ICN2) in Barcelona and PLATON group at the Instituto de Nanociencia y Materiales de Aragón (INMA) in Zaragoza has recently demonstrated that MOPs with alkyl chains covalently attached to the ligand backbone can form stable monolayers at the air−liquid interface where they can be densified and further transferred to solid substrates, providing access to dense and ultrathin films of interest for membrane development.
In their last study, the same research groups expand the fabrication of Rh-MOP films to different compounds with aliphatic chains attached on different sites of the compounds (Figure 1). These chains can be incorporated ex-situ before spreading Rh-MOP onto the liquid surface or in-situ at the air−liquid interface. Moreover, an acid treatment after film formation allows a partial ligand cleavage, which modifies the hydrophobicity of the film surface, opening post-synthetic modifications of the properties of ultrathin MOP-based films.
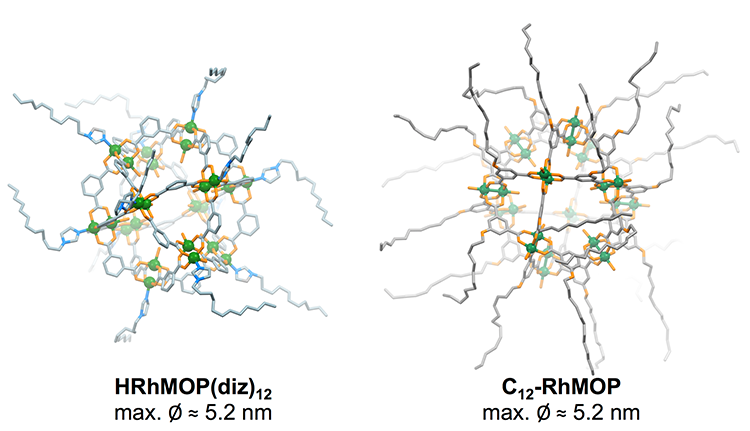
Figure 1: The two types of functionalized Rh-MOPs and their estimated size: with 12 alkyl chains through coordination of an imidazole ligand at each Rh pair (left, HRhMOP(diz)12) or 24 alkyl chains covalently anchored on the organic ligand (right, C12RhMOP).
In-situ Grazing Incidence X-ray Diffraction (Wide Angle – GIXD) and Grazing Incidence Small Angle X-ray Scattering (GISAXS) data obtained at the SIRIUS beamline allowed to determine the structure of the films on water (figure 2). The alkyl-functionalized Rh-MOP molecules form a sort of glassy film at the air−water interface, with the Rh-MOPs maintaining motional degrees of liberty, thus having variable orientations and inter-MOP separations. The film is formed at rather low pressure, probably as soon as inter-MOP interactions are favored through interdigitation of the alkyl chains. Although the chains show some in-plane order, this does not result in a regular organization of the Rh-MOPs in the film.
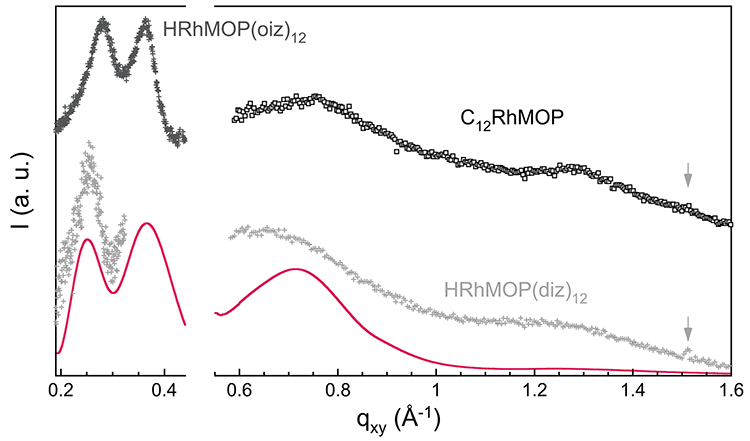
Figure 2: In situ GIXD and GISAXS data for Rh-MOPs at the gas−water interface. The red line is the diffusion form factor of core−double shell spheres with the MOP characteristics. Vertical arrows highlight a peak characteristic of alkyl chain ordering.
Mixed membranes were then fabricated by transferring multiple layers of these glassy Rh-MOPs films onto a permeable polymer and their CO2/N2 separation performance in post-combustion conditions1 were determined. All multilayered films, including those obtained after in-situ interfacial modification, showed efficient CO2 separation, comparable or even better than that of polymers with intrinsic microporosity (PIMs) – PIMs are highly permeable polymers, intensively studied, that are very promising for gas separation processes.
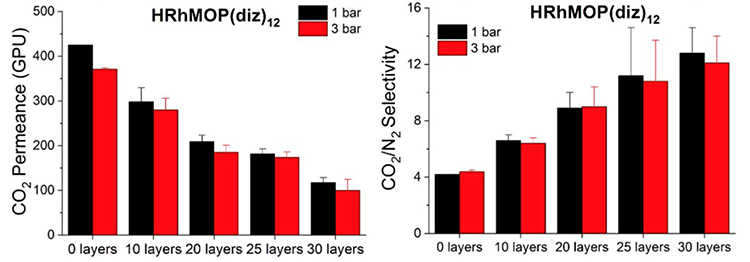
Figure 3: CO2 permeance and CO2/N2 selectivity measurements at 35ºC of HRhMOP(diz)12 / permeable polymer (PTMSP) membranes with increasing number of Rh-MOP layers.
Overall, the study has expanded the range of compositions of MOP films, including previously inaccessible MOPs (i.e. insoluble MOPs), while demonstrating the possibility of post-synthetic modifications, and giving access to the fabrication of MOP-based membranes with tailorable and competitive performance in CO2/N2 separation.
1 - In power plants, fuel is mixed with air and burned. The heat released produces steam, which is used to drive the turbine that produces electricity. The post-combustion gases consist mainly of nitrogen (from air) and lower concentrations of water vapor and CO2. This is why the ability of MOPs to capture CO2 is studied in post-combustion conditions: comparable to the conditions existing after combustion of gas, coal or biomass; in this study the separation of CO2 was carried out using a mixture of 10% CO2 and 90% N2, at 35°C and at 2 different gas pressures (1 and 3 bars).