The conversion of methanol-to-hydrocarbons is an attractive catalytic technology to obtain gasoline or olefins from alternative feedstocks, such as natural gas and biomass. This process is catalyzed by acidic materials, better known as zeotypes, which are going to define the selectivity to the desired product.
The methanol to hydrocarbons reaction is a very dynamic system where it is believed that methylated aromatic species encapsulated in the structure of the catalyst are the main engine of the reaction. At the same time, those aromatics can be the precursors of more poly-aromatic species, which plug the pore system and therefore deactivate the catalyst. The industrial application of this technology will depend on the design of novel advanced materials with a better performance, which requires sophisticated tools to understand the chemistry and position of those aromatic species.
A collaboration between scientists from Utrecht University and the SMIS beamline has made possible to understand to a greater degree the chemistry and location of those aromatic species in individual micron-sized catalyst particles during the methanol-to-hydrocarbons reaction. The high brilliance of the beam makes it possible to probe not only individual catalyst particles, but also their intra-particle distribution.
The results show that in materials with chabazite structure, two distinct chemical stages can be observed during the methanol-to-olefins reaction. In the first one small hydrocabons, such as dimethyl ether, methoxy species and methylated mono-aromatics, are created quite homogeneously inside the cages of the material. In the second stage more complex poly-aromatic species are formed at the periphery of single crystals, as illustrated in Figure 1, which block the porosity and deactivate the material.
Additional isotopic labelling studies reveal that the species created in the first stage of the reaction are very dynamic and they are not influencing the accessibility of the material. On the contrary, the poly-aromatics species created during the second stage of the reaction are not isotopically exchanged, therefore they are not active during the reaction and deactivate the material.
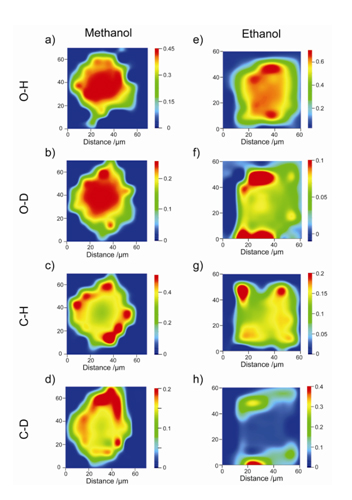
Figure 1. Spatially-resolved chemical maps of single 50 µm-sized SAPO-34 crystals measured after two H/D exchange experiments at 553 K by switching from H4-methanol to D4-methanol at stage I of the methanol-to-olefins reaction (a-d) and by switching from D6-ethanol to H6-ethanol during the ethanol-to-olefins reaction (e-h). The results include intensity maps of the (a & e) O-H stretching vibration, (b & f) O-D stretching vibration, (c & g) C-H stretching vibration and (d & h) C-D stretching vibration, obtained by measuring the intensity of the 3590 cm-1, 2650 cm-1, 2209 cm-1 and 2925 cm-1 bands, respectively. The step size is 15 µm with an oversampling of 7 µm.
By applying the same methodology the chemistry and crystal accessibility has been unraveled in an analogous reaction system, namely the ethanol-to-hydrocarbons reaction. In contrast to methanol-to-hydrocarbons, this reaction consists of one major stage comprising the formation of mono- and poly-aromatics in one step. Consequently, the spatial distribution of the species and accessibility of the crystals is influenced to a great extent. These findings, summarized in Figure 2, have delivered new fundamental insight into the chemistry and deactivation mechanism of these catalytic systems and highlight the importance of studying such materials at the single particle level.
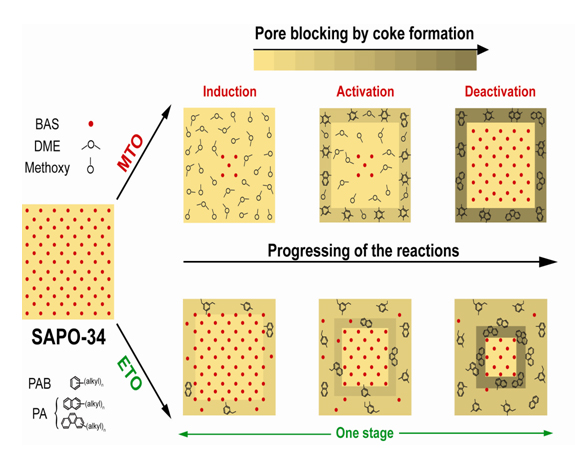
Figure 2. Schematic representation of the evolution of the retained hydrocarbon species and the crystal accessibility in individual SAPO-34 crystals as a function of the reaction stages during the methanol-to-olefins (top) and the ethanol-to-olefins (bottom) reactions at 553 K.