Diatomic molecules are omnipresent in our environment, as well as in the air we breathe, but if we increase pressure by gradually bringing them closer together, how long do we continue to refer to them as differentiated molecules?
An international team from Germany, China and Japan, in collaboration with scientists from Université Lyon 1 and Sorbonne Université, have conducted experiments on SAMBA beamline that demonstrate that, for halogens, the progressive confinement imposed by pressure has a real effect on atom pairs: their bonding evolves, and not in an entirely conventional manner.
The application of pressure leads the gaseous phases of diatomic molecules to condense first into liquid and then into solid phases. In these condensed systems, a certain degree of interaction between the molecules is established, but is essentially weak, of the van der Waals type, preserving the molecular nature of the system. Once the crystal is formed, compression of the solid gives rise to a succession of crystalline phases that progressively reduce the intermolecular space. But what response do these pairs of atoms have to an evolving and increasingly restricted environment? In general, pressure-induced X-ray diffraction studies show an unshakeable stability of the diatomic pair: the atom pairs do not seem to be affected despite increasing confinement... up to the inevitable separation: the dissociation that gives rise to the atomic phase. In the case of halogens, the discovery of an incommensurate modulated phase prior to dissociation had already undermined this conviction. In this phase, an intramolecular distance variation of about 10% opposes the unique value of the low pressure molecular Cmca structure phase.
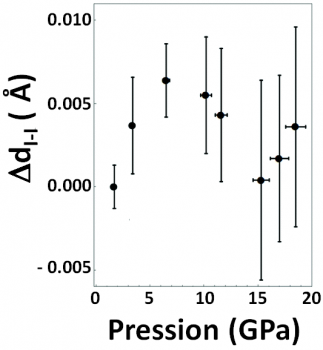
Figure 1: Iodine intramolecular distance variation in the Cmca phase as a result of pressure.
However, 15 years ago, our X-ray absorption spectroscopy studies had already demonstrated that, even in the low-pressure Cmca phase, bromine atoms were not unresponsive to the increasingly close presence of other neighbouring molecules. Our research indicated that, under the effect of pressure, the atoms in the pair separated progressively, but only up to a certain critical pressure, after which the effect of confinement consolidated the pair, which came closer together again. The similarity of the phase diagrams of bromine and iodine had led us to attempt the same type of study on iodine. But it was not as easy as we had initially imagined...
Four measurement campaigns at ESRF, SLS and finally SOLEIL were successively conducted over a period of 15 years, according to the technical developments required, as the heavier iodine followed the same evolutionary pattern as bromine, but with a 10-fold lower molecular distance evolution (see Figure 1). This required a considerable feat of precision that was achieved by combining the stability of the SAMBA beamline with the use of polycrystalline diamond anvils and meticulous data analysis.
Atomistic modelling using DFT (Density Functional Theory) allowed us to understand that, during compression, a network of intermolecular bonds increased the electron density between the molecules in the plane and led to its initial weakening, as shown in Fig. 2.
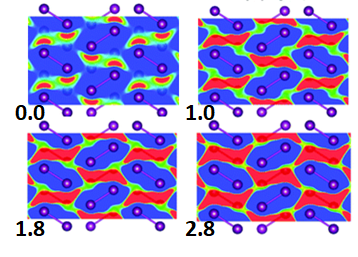
Figure 2: Evolution of the electron density between molecules under the effect of pressure (relative values in GPa indicated in bold) in the Cmca phase obtained by DFT.
In transparency, we can observe the iodine molecules in the lower plane. We can observe an increase in the electron density (denser areas in red, less dense areas in blue) between the molecules which increases above (and below) the molecules in the neighbouring planes.
The change in behaviour of bromine and iodine molecules in the Cmca phase occurs at 25 and 7 GPa respectively. Using scaling laws based on the definition of a reduced molecule volume, we predict a behavioural transition for chlorine at a pressure of 42 ±8 GPa.
It remains to be determined whether this behaviour observed for halogens can be generalised to other pairs of diatomic molecules, some of which, such as hydrogen, have a dominant presence in the universe under extreme pressure conditions.