Copper oxide materials, also known as cuprates, feature the fascinating ability to become superconducting below a critical temperature Tc. Understanding the collective electron properties in cuprates is a major scientific challenge in the quest of enhancing Tc to room temperature, which would amount to a technological revolution.
An international consortium led by researchers in Palaiseau, Paris, and Grenoble combined theory and experiments at SOLEIL CASSIOPEE beamline to explain how magnetic fluctuations modify the cuprates’ electronic properties in vicinity of the superconducting regime.
Superconductivity in cuprates, and most other high-temperature superconductors (HTSCs), is a consequence of the strong correlations between electrons. The latter cannot be described as individual entities, but rather as collective states emerging from the interactions between them. Such behavior is directly visible in the distribution of electronic states of cuprates and other HTSC. One of their footprints is an anomaly called “waterfall”, in reference to the peculiar shape of the electronic dispersion, see Fig. 1. Understanding its origin would be a crucial leap forward since it is universally found in cuprates and affects the very electrons that become superconducting at low-enough temperatures. Yet, the origin of the waterfall and so-called kink features, in particular the corresponding quasiparticle(s), remains debated.
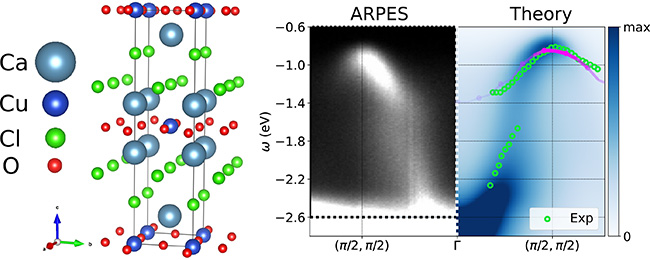
Figure 1: Na-CCOC crystal structure, and comparison between ARPES (from Ronning et al., PRB 71, 094518 (2005)) and theory (color plot and purple curve) for an undoped sample. The green circles are extracted from the measurements.
To address this issue, the team systematically compared state-of-the-art angle-resolved photoemission spectroscopy (ARPES) measurements carried out on the representative cuprate NaxCa2-xCl2CuO2 (Na-CCOC) with theoretical calculations. High-resolution ARPES experiments were performed on hole-doped samples at SOLEIL’s CASSIOPEE and Photon Factory BL-28 (Japan) beamlines, and complemented with data for undoped samples from the literature. The researchers constructed and solved a material-specific theoretical model without hand-tunable parameters, in which the electrons were coupled together by coherent magnetic fluctuations, so-called magnons.
Demonstrating nearly perfect agreement between theory and experiments, it provides an interpretation of the waterfall and kink features, which constitutes the key message of the present work: In cuprates, the electrons strongly couple to the magnetic fluctuations and form “spin-polarons”. This finally translates into the waterfall and kink anomalies in the distribution of electronic states. Most notably, the strength of the coupling depends mainly on the direction of propagation of the electrons, rather than their energy. This explains why the waterfall and kink features appear at different characteristic energies in the undoped (insulating, Fig. 1) and hole-doped (metallic, Fig. 2) cuprates.
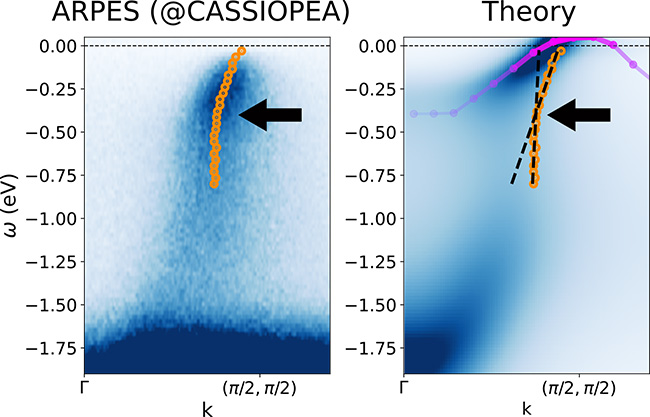
Figure 2: Comparison between ARPES and theory (color plot and purple curve) for a 10%-doped sample. The orange circles are extracted from the measurements.
The main result of this study, a description of the spectral properties of the electrons becoming superconducting at low-enough temperature, has direct consequences for our theoretical understanding of high-temperature superconductivity. For superconductivity to emerge, electrons form Cooper pairs via an effective attractive interaction. Undoubtedly, the present interpretation of ARPES spectra shows that magnetic fluctuations should play a central role in the pairing of electrons, and thus motivates further work for establishing its precise microscopic mechanism.
Future measurements extending the present study to larger values of hole-doping, which amount to the optimal doping regime for superconductivity, are needed to provide a complete description of the fate of spin-polarons in high-temperature superconductors.