In order to deal with the recent decline in the effectiveness of antimalarial treatments, new therapeutic solutions are currently being explored to fight against malaria. Researchers from University Toulouse 3, the Servier Laboratories and the company Vectalys studied synthetic molecules (INODs) known for their antimalarial activity. Despite the fact that the mechanism of action remains to be elucidated, the Quinone reductase 2 (hQR2) was early suspected to be the main target of INODs antimalarial compounds. 3D structures of INODs/hQR2 complexes were determined by X-ray diffraction studies performed at the PROXIMA 1 beamline. These crystallographic structures, combined with kinetic and EPR experiments, help understanding why INODs are effective in the treatment of malaria.
These unexpected results, published in the Molecules journal, opened exciting research perspectives to explore a new class of antimalarial compounds.
Malaria is a life-threatening infection occurring in tropical and subtropical countries. This disease is transmitted through a bite from a mosquito infected with the one-celled Plasmodium parasite. In the past two decades, broad use of antimalarial medications associated with insecticide-treated nets and indoor sprays helped reduce malaria mortality rates by 62%. However, resistance to all classes of antimalarial drugs is responsible for a recent increase in malaria-related mortality and has raised the need to identify novel therapies.
When the parasite adapts to treatments...
Four species of plasmodium are known to infect humans, but one, Plasmodium falciparum, accounts for over 400.000 deaths per year. During the liver stage of the infection cycle the parasite enters the blood stream and infect the liver cells. When the infection is untreated the parasites maturate and infect erythrocytes (red blood cells) during the erythrocytic stage and can lead to death.
Over the past five decades, routine treatment of malaria relied on combinations of inexpensive and widely available quinoline-type schizontocides (i.e. molecules that kill the parasite when it becomes active) such as the 8-aminoquinoline (primaquine) and the 4-aminoquinoline (chloroquine). These molecules act at the liver and erythrocytic stages of the infection, respectively. However the extensive deployment of these drugs has resulted in the onset of resistant strains particularly in P. falciparum, accounting for a global resurgence of malaria. Subsequently Artemisinin-based combinatory therapies were introduced in the mid-1990s and resulted in a substantial reduction of mortality from malaria. Recent cases of Artemisinin resistance, however, have been documented and the development of novel rational therapy approaches has become compelling.
Understanding the role of molecules already used in treatments
The quinone reductase 2 (hQR2) catalyzes the two-electron reduction of quinone substrates. Exhaustive studies have highlighted that QR2 is the only human target for quinoline-types of antimalarial drugs that bind and act as inhibitors. As a consequence, strong efforts to synthetize new potent inhibitors of QR2 were provided, believing that these molecules could work as potent antimalarial agents. Unfortunately no link could be established between the inhibitory and antimalarial properties of the new molecules.
Furthermore, indolone-N-oxides (INODs) are pseudo-quinone compounds that display antimalarial activity against Plasmodium falciparum at the erythrocytic stage. Among all the INODs, only molecules that contain a pseudo-quinoid structure and a reducible C=N bond display antimalarial activity, such as INDs obtained from INODs deoxigenation. Although the mechanism of INODs reduction remains unclear, the experiments reported in the Molecules journal show that substrates of QR2 display antimalarial activity. In these studies, kinetic essays showed that QR2 metabolizes INODs at slower rates when compared to the natural substrate menadione providing for the first time evidence that substrates rather than inhibitors of QR2 display antimalarial activity.
X-ray diffraction data collected at PROXIMA-1 then allowed the structure determination of hQR2 in complex with flavin adenine dinucleotide (QR2-FAD) and bound to an INOD and an indolone selected subsequently to the enzymatic tests, compound 8′ or compound 10, respectively. These structures confirm the recognition of the compounds by QR2, highlighting their binding geometry in the catalytic site of QR2 above the FAD, as previously observed for other substrates and inhibitors of hQR2. Compounds 8′ and 10 establish attractive and non-covalent interactions with the isoalloxazine ring of the FAD and did not interact directly with the protein residues (Fig.1).
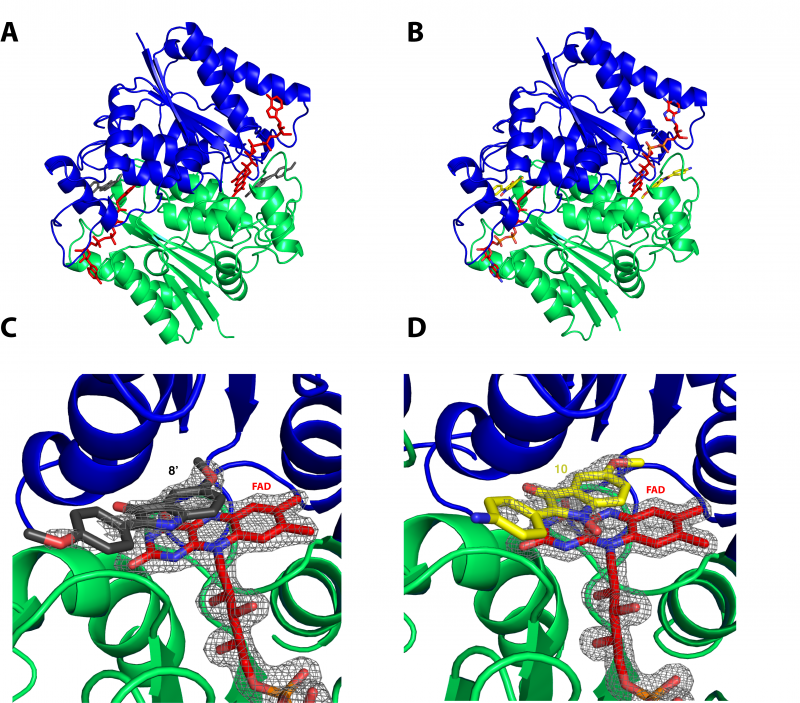
Figure 1: (A,B) Overall architecture of human quinone reductase 2 (hQR2) bound to the coenzyme flavin adenine dinucleotide (FAD) and to compound 10 or compound 8′ respectively. Crystals of these complexes belong to the space group P 21 21 21, with two molecules per asymmetric unit. (C,D) Electron density surrounding FAD and compound 8′ or compound 10 (red, grey and yellow sticks respectively) show that the ligand could only be partially modeled likely due to its flexibility and exposure to the solvent.
The antimalarial activity is not as expected
Quinones are known to generate free radicals upon reduction by QR2. In this study, EPR measurements were performed on indolone derivatives in the aim of emphasizing possible radical formations upon reduction by QR2. Interestingly, indolone derivatives reduced by hQR2 also produce free radicals. However, indolone derivatives that do not possess a pseudo-quinoid structure cannot be metabolized by QR2 and do not display antimalarial activity, suggesting a tight link between the production of radicals upon reduction by QR2 and the antiplasmodial activity of these compounds. Similar experiments were conducted on healthy and parasitized RBC and on native and QR2 transfected CHO cells and demonstrated that the production of radicals varied depending on the levels of QR2 expression and on the nature of the substrate tested and its IC50 value on P. falciparum (Fig.2)
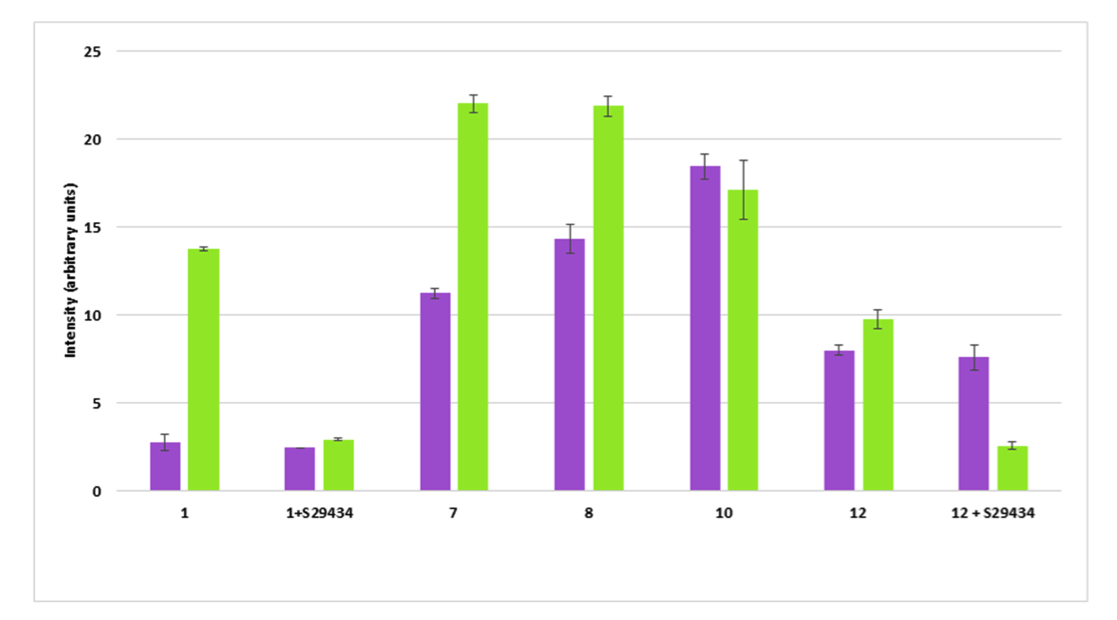
Figure 2: The addition of indolone-N-oxide antimalarial compounds in the CHO-QR2 transfected cell line overexpressing QR2 (orange bars) led to more intense EPR signals than in the CHO-NT native cell line expressing endogenous QR2 (blue bars). Addition of the QR2 inhibitor S29434 significantly reduced the intensity of the signals. The most active compounds (compounds 7 and 8) lead to the highest EPR signal intensities. These results confirmed the role of hQR2 in the reduction of the indolone-N-oxide compounds and in the production of radicals responsible for their antimalarial activity.
Up to date, the antimalarial properties of indolone-N-oxide compounds were ascribed to their ability to inhibit hQR2. Yet, the crystal structures of hQR2 bound to indolone-N-oxide compounds combined with kinetic and EPR measurements show that hQR2 reduces INODs and indolones giving rise to unstable compounds that tent to re-oxidize and produce free radicals that are fatal for parasitized RBCs. These results reveal that substrates of hQR2 rather then inhibitors represent a new class of promising molecules against malaria.