SVOM (Space based multi-band astronomical Variable Objects Monitor) is a Sino-French space mission to be launched in 2023 to observe gamma-ray bursts. The on-board Microchannel X-Ray Telescope (MXT) provides the positions and the time-resolved spectra of the soft X-rays (0.2-10 keV) emitted after the gamma-ray flashes. The flight spare detector of the MXT was the object of a calibration test campaign on the METROLOGIE beamline in order to characterize its response and quantum efficiency at such energies. The beamline adjustments were deeply adapted to respect the detector constraints. The main challenge was to reduce the photon flux of the beamline by 10 million times, while preserving the beam spectral purity.
Gamma-ray bursts are brief flashes of gamma rays, appearing in random directions in the sky. They are produced by the explosion that follows the gravitational collapse of massive stars or the coalescence of two neutron stars members of binary systems. After this initial flash of gamma rays, a longer-lasting 'afterglow' is usually emitted at longer wavelengths (up to several months): X-rays, ultraviolet, visible, infrared, microwave and radio waves. The SVOM satellite mission will embed four instruments to study these violent phenomena. ECLAIRs and GRM (Gamma-Ray burst Monitor) with their large fields-of-view will detect and roughly localize these bursts in the gamma-ray range, triggering a satellite maneuver towards their direction. The narrow field-of-view MXT and VT (Visible Telescope) instruments will then provide a precise burst position and observe their fading counterparts in the X-ray and visible light, respectively.
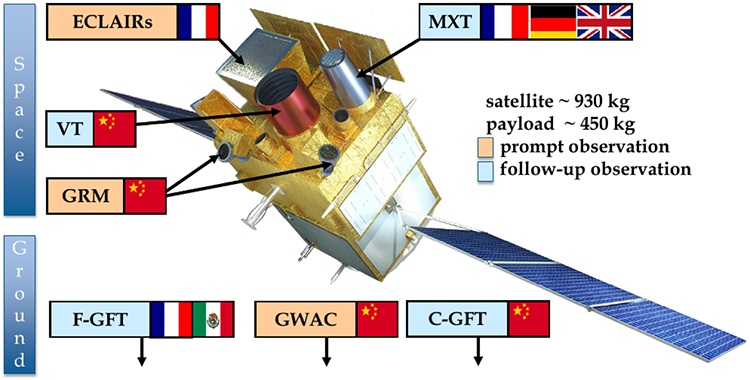
Figure 1: Schematic of SVOM showing the four instruments embedded for the mission
The Microchannel X-ray Telescope is under CNES (French space agency) responsibility. It was designed and realized by CEA-Irfu, CNRS-IJCLAb, the University of Leicester and a set of European companies. The camera includes a 19.2 x 19.2 mm² charge-coupled device (pnCCD), provided by the Max Planck Institut für Extraterrestrische Physik. The flight model was delivered to CNES in May 2021. The flight spare, produced from the same wafer as the flight one, is kept at CEA-Irfu for complementary characterizations.
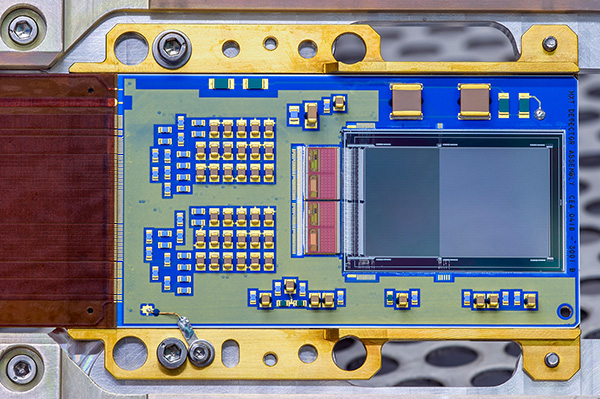
Figure 2: Detector of the MXT telescope, a 'spare' of which was tested on METROLOGIE beamline
Experiment
The measurements were performed in the 0.3-1.8 keV energy range, corresponding to the range where most of the photons are collected in the MXT. At low energy, the detection efficiency of the telescope is limited by the visible light filter deposited on the detector chip itself, necessary to avoid optical contamination when looking at X-ray sources. The detection chain is designed for the very low fluxes of astrophysical sources. Consequently, the photon flux used to test the detector should be of the order of 1 photon/s/pixel (with pixels of 75 x 75 µm²) to avoid photons pile-up (the detector is read with a rate of 10 frames per second). But the METROLOGIE beamline typically delivers 109 to 1010 photons/s in a focal spot of 200 x 100 µm². Thus, the challenge of the SOLEIL and CEA teams was to deeply attenuate this flux while keeping the spectral purity of the beam.
The detector was placed 5 m away from the focal spot-where the photon density is highest- to reduce the flux in each pixel, taking advantage of the beam divergence. X-ray filters were placed in the beam to strongly reduce the quantity of photons deposited on the detector. Even in this low-flux configuration, the beam spectral purity could be maintained at better than 99% by eliminating photons that did not have the desired energy using a so-called low-pass filter system.
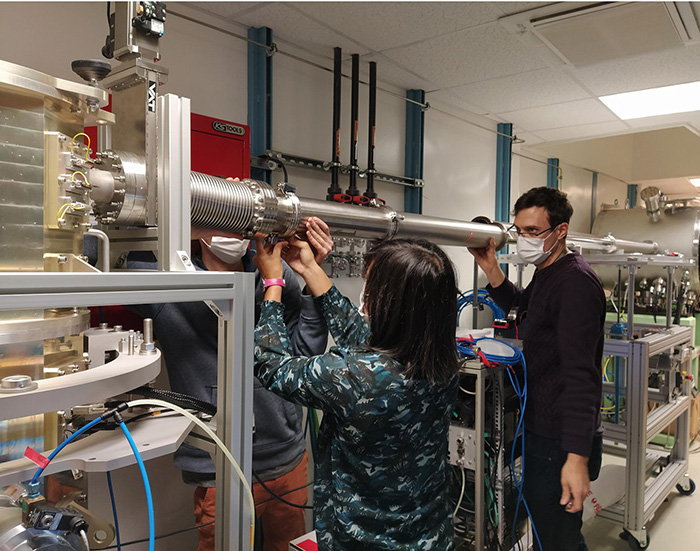
Figure 3 : Experimental setup on the METROLOGIE beamline. The cryostat housing the MXT (far left of the photo) is placed 5 m from the focal spot to illuminate 4 % of the detector area (3 mm x 5 mm, ~2500 pixels). Inside the cryostat, the detector is cooled down to -65°C.
Results
Functional beam configurations were found for different energy bands, with an excellent spectral purity and no pile-up in the detector - a flux of 1 photon/s/pixel should be aimed for: each pixel should be impacted by only one photon of a given energy; here, less than 1 % of the photons was measured at twice the energy. The photon flux was of the order of 150 photons/s.
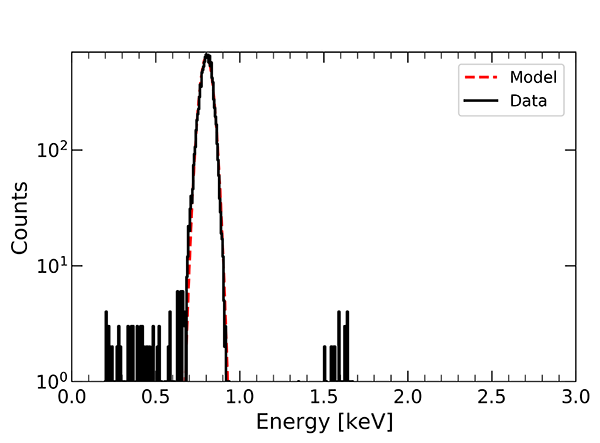
Figure 4: Example of spectrum measured by the detector at 800 eV with a photon flux of 150 photons/s (1 photon per pixel; less than 1% of the photon is measured at twice the beam energy). The results obtained (in black) are as expected in the model (in red).
Excellent measurements of the transfer function “eV to ADU” (conversion of the signal measured by the detector, in V, to an energy value, eV), the energy resolution of the detector chain, and the CCD charge transfer efficiency were obtained. To measure the quantum efficiency of the detector (ratio between the number of events detected and the number of photons actually received by the detector), the absolute incident flux on the detector must be calculated from high-flux measurements given by an absolutely calibrated X-ray photodiode. This indirect measurement is much more challenging and requires additional calibration measurements that are foreseen in a second campaign.
Conclusions
This first campaign was a great technical success in operating the METROLOGIE beamline in an unusual low flux regime. This offers perspectives for the characterization of photon counting devices with high spectral performances in the X-ray range. The CEA team is preparing technical improvements of the experimental setup for a second test campaign, working in close collaboration with the SOLEIL team to improve metrology capabilities in this very-low photon flux regime.