During the development of a wheat grain, the grain’s cells synthesize storage proteins, which assemble into dense, spherical structures called protein bodies. These protein bodies act as stable storage of carbon, sulfur, and nitrogen for the plant's growth during germination. Despite numerous studies, the exact mechanism of protein body formation remains unknown. Scientists from INRAE - Nantes have investigated this question using an in vitro microfluidic technique on the DISCO beamline to study γ-gliadin, a key wheat storage protein.
The formation of protein bodies was initially attributed to the spontaneous precipitation of wheat storage proteins due to their low solubility in water. Subsequent in vivo studies revealed that protein bodies are dynamic structures that undergo fusion and exchange their content overtime. In vitro research demonstrated that wheat storage proteins can undergo liquid-liquid phase separation, suggesting that this process could be involved in the early stages of protein body formation. However, uncertainties remain about the compatibility of this phase separation with biological conditions. Additionally, the endoplasmic reticulum* membrane could play a crucial role by serving as an anchoring point for protein assembly through protein-membrane interactions, particularly due to gliadins', essential wheat storage proteins, high affinity for lipid membranes.
The study aimed to explore the liquid-liquid phase separation of wheat gliadins under aqueous conditions close to biological conditions and to characterize a potential competition with protein-membrane interactions. To this end, a microfluidic approach (figure 1) was developed to generate giant semi-permeable lipid vesicles, which act as microreactors where physicochemical conditions can be precisely controlled. The scientists examined the influence of pH and gliadin concentration on these vesicles and the interactions between gliadins and vesicle membranes.
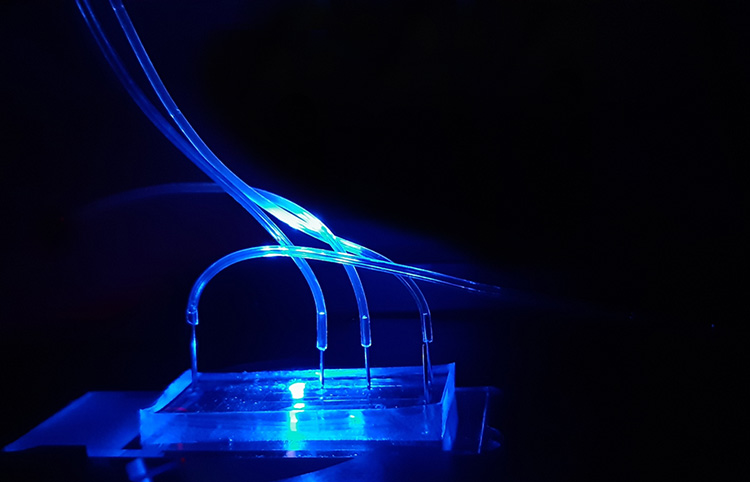
Figure 1: Photo of the microfluidic setup used for the experiment
For this purpose, autofluorescence microscopy was used on the DISCO beamline to detect the presence of proteins on the membrane surfaces. Additionally, phase-contrast microscopy, confocal laser scanning microscopy (CLSM), and fluorescence spectroscopy were employed to study the microstructure of protein assemblies depending on pH and gliadin concentration.
The scientists demonstrated that γ-gliadins encapsulated in vesicles can undergo phase separation induced by pH through two distinct mechanisms, depending on protein concentration. At low concentration, γ-gliadins separate by a nucleation and growth process, while at higher concentrations, they form a bicontinuous phase suggesting a mechanism called spinodal decomposition (see Figure 2). In all conditions, γ-gliadin assemblies localize either on the membrane surface and/or settle at the bottom of the vesicles.
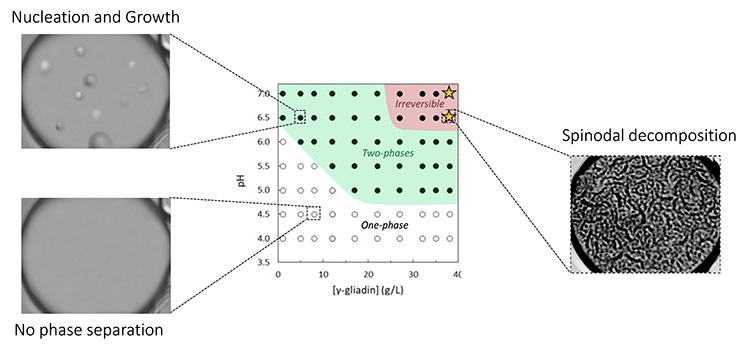
Figure 2: Phase diagram of γ-gliadin encapsulated in semi-permeable vesicles as a function of pH and gliadin concentration. The white area of the diagram indicates a monophasic system, while the green area indicates a reversible biphasic system, and the red area indicates an irreversible biphasic system. Homogeneous system conditions are marked by empty symbols, while filled symbols indicate phase separation. Conditions leading to nucleation and growth are distinctly marked by black circles and spinodal decomposition by yellow stars.
To study the microstructure and localization of γ-gliadin in the vesicles, experiments were conducted using CLSM and UV autofluorescence microscopy on DISCO. In CLSM, gliadins labeled with a fluorescent dye visible under light (orange color) were encapsulated under good solubility conditions (pH 4.0). By increasing the pH to 7.0, phase separation was observed in colocalization with the lipid membrane, suggesting an affinity of the dense phases for the membrane (Figure 3). The intense fluorescence observed on the membrane surfaces persisted even after returning to pH 4.0, suggesting partial irreversibility of the phase separation.
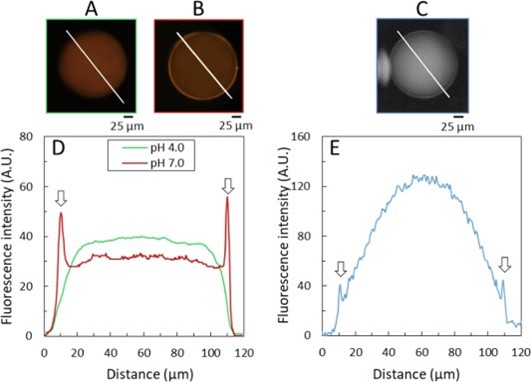
Figure 3: A & B. Images obtained by confocal laser scanning microscopy of a vesicle encapsulating γ-gliadin at 10 g/L at pH 4.0 (A) and pH 7.0 (B). C. UV autofluorescence image of a vesicle encapsulating γ-gliadin at 5 g/L and pH 4.0. D. & E. Fluorescence intensity profiles along the white lines shown in images A, B, and C, respectively. Arrows indicate fluorescence intensity peaks at the membrane interfaces.
Complementary UV autofluorescence microscopy experiments were conducted to avoid using fluorescent probes – indeed, gliadins naturally fluoresce when illuminated by UV light. Even under good solubility conditions, a fluorescent layer at the GUV interfaces was observed, suggesting, like the CLSM results, an adsorption of γ-gliadin on the membrane.
These new experiments provide better insights into the biological context of protein body formation in wheat grains. In particular, the study confirms the occurrence of phase separation in the presence of lipid membranes and aqueous environments. The established phase diagram shows a very low saturation concentration at pH 7.0, suggesting that a low local concentration could be sufficient to generate protein bodies during gliadin synthesis. Although the biological complexity of seed development is not yet achieved with this experimental protocol, phase separation remains a promising avenue to understand protein body formation in seeds.
*Endoplasmic reticulum: a cell compartment, bounded by a membrane, where proteins are synthesized.
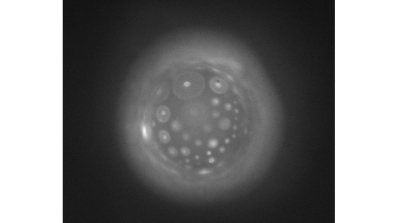
Illustration photo: Gliadin body adsorbed on an oil droplet - autofluorescence microscopy image obtained on DISCO