A Swedish team provides key insights with a new 3D structure, solved from data collected on PROXIMA1, of the xylose transporter XylE from E. coli.
Researchers from Karolinska Institutet in Stockholm have obtained diffraction data on the PROXIMA1 beamline that enabled the structure determination of the xylose transporter XylE from Escherichia coli in an inward open conformation. This result verifies the long standing ‘alternate access’ hypothesis for the transport mechanism of this class of proteins and demonstrates that the two domains of the protein move relative to each other by substantial rigid body movements during the functional cycle. These results are published in the journal Nature Structural and Molecular Biology.
Transport over the plasma membrane: the MFS mission
Selective transport of ions and small organic compounds over the plasma membrane is essential to maintain homeostasis in all cell types. This task is carried out by a plethora of channel and transporter proteins. One of the largest transporter classes is the major facilitator (MFS) superfamily, which is found in all branches of life and has diversified into numerous families with different substrate specificities. In MFS transporters, the substrate binding site is localized between the two domains of the protein, and it is believed that transport occurs by an ‘alternate access mechanism’ where the protein can be either in an outward open state (binding site only accessible from the extracellular environment), occluded state (binding site inaccessible) or inward open state (binding site only accessible from the cytoplasm). Thus, according to this hypothesis, substrate binding in the outward open state is followed by a conformational change to the occluded state, which prevents the substrate from leaving the binding site, and this is subsequently followed by another conformational change to the inward open state, which allows the substrate to be released, i.e. imported, into the cytoplasm.
GLUT for the mammalian glucose…
The most well-known MFS family is probably the mammalian glucose transporter (GLUT) family. Here GLUT1-4 have been particularly well studied: GLUT1 is responsible for basal glucose uptake in most cell types and is highly upregulated in many cancer forms, GLUT2 is essential for regulation of insulin secretion, GLUT3 is together with GLUT1 responsible for glucose uptake in the central nervous system, and GLUT4 is the insulin responsive glucose transporter of muscles and adipose tissue (body fat).
In order to understand the transport mechanism of MFS transporters in general and of GLUT transporters in particular, researchers from Karolinska Institutet in Stockholm undertook to crystallize a bacterial homologue of the mammalian GLUT family –the xylose transporter XylE from Escherichia coli.
… and XylE for the bacterial glucose
Using diffraction data obtained at PROXIMA1 beamline, the scientists were able to determine the crystal structure of XylE in an inward open conformation (Fig. 1A). By comparing this structure with two other structures of XylE; partially occluded inward open (diffraction data obtained in Diamond, results also published in this article), and partially outward open occluded (ref.1) (Fig. 1B), they could conclude that XylE functions by an alternate access mechanism and that transition from the partially outward open occluded to the inward open state involves a substantial rigid body tilt movement of the two individual domains (Fig. 1C).
What controls these movements? It appears that control of the structural changes that underlie the functional cycle is achieved in different ways in different MFS families. However, it is clear that a set of highly conserved cytoplasmic ‘signature residues’ are essential for this task in the mammalian GLUT family. These signature residues are also present in XylE and when comparing the three available structures, it becomes clear that the breaking and reformation of a number of ionic interactions between these residues are critical for controlling access to the binding site from the cytoplasm.
Conclusion
XylE is the first MFS transporter that has been determined in more than one conformational state. These results thus provide the first direct structural evidence for the validity of the alternate access hypothesis for a MFS transporter, and open up unprecedented opportunities for further understanding of the transport mechanism through computer simulation studies (molecular dynamics). Furthermore, these data highlight the importance of the GLUT signature residues in controlling the rigid body movements that open and close the access to the binding site from the cytoplasm.
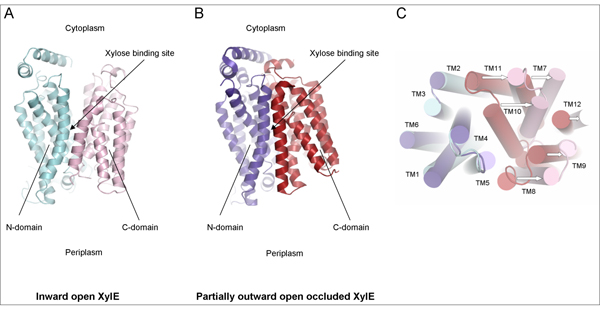
Figure 1. Structural differences between the inward open and occluded states of XylE. A. Side view of the inward open state of XylE. B. For comparison, the structure of XylE in the partially outward open occluded state is shown in same orientation. C. The structures shown in A and B were overlaid. They are colored in the same way, but a different representation style was used for the transmembrane helices and the structures are here viewed from the cytoplasmic side. The transmembrane helices (TM) are labeled and arrows designate changes in the position of the helices upon opening of the transporter towards the cytoplasm: The two domains move as rigid bodies upon opening/closing.
Reference 1: “Crystal structure of a bacterial homologue of glucose transporters GLUT1-4”. Sun, L. et al. (2012). Nature. 490:361-366.