"Multifunctional" materials have several physical properties that can be used in a single application. The coupling between their different physical properties is one of the key questions to address in order to understand their operation in the perspective of future developments of these materials. Magnetoelectric multiferroics are an excellent example of multifunctional materials. Their properties originate from the interdependence between their elasticity and their ferroelectric and ferromagnetic properties. The beamlines DEIMOS, SIXS and DIFFABS, which are particularly well suited to the simultaneous determination of all these characteristics, even using very small quantities of material, have allowed the researchers of CEA/SPEC, INSP, SOLEIL and IMPMC to study their interactions.
Magnetoelectric coupling is generating a high level of interest in the range of applications linked to spintronics and the conversion of energy. Strong couplings make it possible to control magnetic properties using an electrical potential, the inverse effect is also possible although technologically less relevant. Conversely, weaker couplings could be used for multi-state magnetoelectric memories: in theory, the use of such memories would allow to multiply the number of states recorded beyond a simple information ‘bit’ (2 states, 0/1). Beyond the increase in information density, multi-state logic would considerably increase computing powers. Unfortunately, the physical principles behind ferroelectricity and ferromagnetism are antagonistic and magnetoelectric components existing at ambient temperatures are rare.
The use of thin film epitaxial layers provides the missing degree of freedom essential for the coexistence of long range magnetic and ferroelectric ordering, and paves the way for the preparation of artificial multiferroic materials. Materials that are highly stable under atmospheric conditions, such as oxides, with high ordering temperatures1, greater than ambient, are ideal candidates. In these systems there is a strong interdependence/interaction between the magnetic, ferroelectric and structural parameters. A relevant study must therefore cover all of these aspects.
This challenge was overcome within the framework of the ANR-IOBTO project consortium (partners CEA/SPEC, SOLEIL and INSP). Using molecular beam epitaxy, the researchers produced heterostructures based on thin ferroelectric layers of barium titanate, BaTiO3 and thin layers of ferromagnetic cobalt ferrite: CoFe2O4. A matrix of samples of reciprocally variable thickness was prepared. Each sample was analysed with respect to its strain states2, its magnetic and electrical properties, using, in particular, a large number of synchrotron radiation techniques to compensate for the small quantity of material in the layers. Indeed, near complete structural relaxation is found when the total thickness of the layers is larger than 30 nm. Thus, very thin layers (below 30 nm) have to be used in order to benefit from the potential of the corresponding magnetoelectric properties.
All of the investigated heterostructures are epitaxial and of excellent crystalline quality (figure 1a). Based on the reciprocal space maps measured using grazing incidence X-ray surface diffraction technique on DIFFABS and SIXS, the researchers were able to extract the meaningful structural parameters and provide evidence of the presence of both a strained and relaxed part in the BaTiO3 layer. In a counter-intuitive manner, it appears that the structural relaxation of each layer depends closely on the total thickness, with an evolution through characteristic steps, starting from adaptation to the substrate for the thinnest layers and extending to total relaxation of each layer (figure 1b).
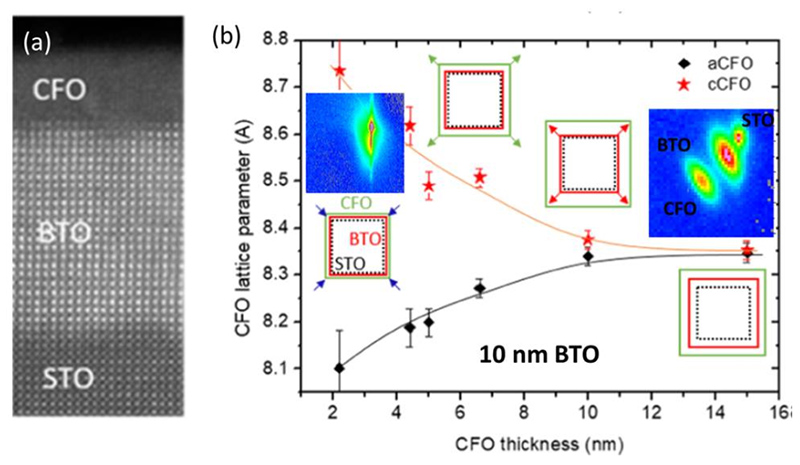
Figure 1. (a) High resolution electron micrograph of a bilayer system CoFe2O4/BaTiO3 deposited on SrTiO3(001). (b) Relaxation of the unit cell parameters as a function of the thickness of the the CoFe2O4 (CFO) layer. The strain state of the BaTiO3 layer is sketched by the insets. For very thin layers, all the in-plane unit cell parameters are stained to the substrate parameter (left-side inset and reciprocal space map around 222 diffraction peak). For the thick layers, the structures are relaxed and the diffraction peaks are perfectly separated in reciprocal space (right-side inset and associated reciprocal space map). In the intermediate situations, the CFO layer relaxes progressively before causing a relaxation of the underlying BTO layer.
In parallel, using X-ray absorption and circular dichroism measurements on DEIMOS, the researchers showed that the chemical environment and the magnetic moment carried by the ferrite unit cells evolved in the same range of thickness, revealing as well a chemical reduction at the interface. Finally, similar to the structural relaxation, the ferroelectric properties of the BaTiO3 are affected by the larger thickness of the ferrite which, when increasing, tends to an improved stabilization of the ferroelectricity (figure 2) and easier electric polarization reversal.
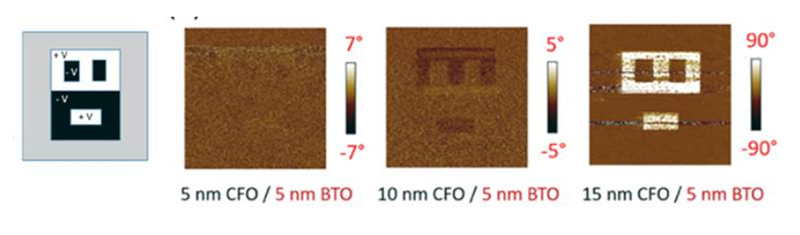
Figure 2. PFM (Piezo-Force Microscope) images, in phase contrast, for a pattern represented on the left-panel after application of the electrical potentials of +/- 8V. Ferroelectric responses for a layer of 5 nm of BaTiO3 (BTO) covered by respectively 5, 10 and 15 nm of ferromagnetic CoFe2O4 (CFO).
In the heterostructures based on CoFe2O4 and BaTiO3, the structural relaxation, the magnetic and ferroelectric properties are therefore not only interdependent (by coupling) but dependent on the individual thicknesses thanks to the elasticity of the structural relaxation of the different layers.
These studies pave the way to the manipulation of the magnetoelectric coupling by strain engineering via simple control of the relative thicknesses of the layers, for a given pair of materials. The choice of the strength of the coupling should permit, starting from the same materials, and by decreasing coupling: (i) a control of the magnetic state of the heterostructure with an electrical potential; or (ii) obtaining of a multi-state element; or indeed (iii) creation of a ferroelectric reference layer frozen in a given state.
[1] Ordering temperature: temperature up to which the long range ordering, whether it be magnetic or ferroelectric, is maintained.
[2] If the thin layer adopting a unit cell parameter close to the ‘ideal’ parameter (relaxed, found in the equivalent monocrystal), or the influence of the substrate (or other encapsulation layers) is strong enough to deform the unit cell of the thin film and ‘impose’ a unit cell parameter possibly closer to that of the substrate.