Recent numbers regarding the climate change are alarming. They call for an urgent change in our energy production in order to reduce our carbon emissions that are responsible for the greenhouse effect. This necessitates the rapid development of alternative energies to hydrocarbon sources: the sustainable energies. To store and transport them, we need “energy vectors”, such as dihydrogen.
In order to improve the efficiency of the dihydrogen production systems, German and French researchers worked on iridium nanoparticles, involved in the catalysis of the water splitting reaction in H2. This reaction could be followed "live" on the TEMPO beamline.
At the Paris Convention in 2015 an international climate agreement, signed by 146 countries, set as a target to limit global warming to 1.5°C by 2050 and 2°C by 2100. These deadlines necessitates the rapid development of alternative sources of energy to hydrocarbon sources. Photovoltaics, wind turbines, and hydro turbines are the most promising for reducing greenhouse gases.
But an urgent and primordial question is the transformation of these renewable energies into an intense energy allowing their storage and transport. Dihydrogen is one of these energy vectors. Its production by electrolysis of water is an interesting and particularly clean solution, producing only oxygen and hydrogen. Electrolysis cells based on proton exchange membrane (PEM) have lot advantages as power flexibility, high current density, safety. However, they require the use of noble metals, Pt cathode and Ir anode, the latter being one of the most rare and expensive. Therefore, considerable research is currently being conducted to reduce the amount of iridium at the electrolyser anode of the PEM type.
Recently, researchers from the German Aerospace Center (DLR) and from Strasbourg University have succeeded in synthesizing very high purity IrOx nanoparticles, improving the activity of the oxygen evolution reaction at the anode (OER) up to 100 A.g-1 to 1.51 V (relative to standard reference hydrogen electrode), which is the highest value reported to date.
To obtain those nanoparticles of very high purity, the researchers have developed an original synthesis method, playing on the quality and the concentration of the chemicals precursors and surfactants used during the synthesis. The nanoparticles obtained were first characterized by Scanning Electron Microscopy (SEM) and Transmission Electron Microscopy (TEM) and then the activity was quantified by cyclic voltammetry.
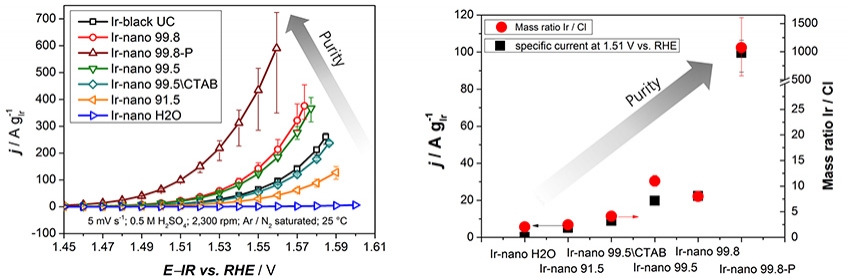
Figure 1: Left- OER activity compared of the nanoparticles. The most active catalysts are those synthesized in ultrahigh purity condition.
Right: Specific current density of the nanoparticles as a function of the purity of the preparation.
The monitoring of this activity was measured at the SOLEIL synchrotron facility with near-ambient pressure photoemission spectroscopy performed on the unique NAP-XPS equipment (Near Ambient Pressure X-ray Photoemission Spectroscopy) of Sorbonne Université, installed on the TEMPO beamline. A specific mounting using an assembly of electrodes on the membrane made it possible to follow the evolution of the reaction in operando mode.
For this purpose, a specific assembly has been made comprising the membrane with the two electrodes, covered with Ir nanoparticles on the anode side (working electrode, WE) and on the opposite side the cathode made of dispersed Pt nanoparticles on carbon (Pt/C counter electrode, CE). The measurements in operando mode were performed in the presence of a water pressure of 3 mbar and different applied voltages. The OER activity was monitored with a mass spectrometer, placed in the lens of the analyzer.
The photoemission spectra of iridium 4f level have been decomposed into three doublets comprising the two spin-orbit components 4f5/2 and 4f7/2 separated by 3 eV. Each of these doublets was assigned to metal iridium (4f7/2 at 61.0 eV), IrIV (4f7/2 at 62.1 eV) and IrIII (4f7/2 at 62.8 eV). The intensity of these components plotted as a function of the applied voltage makes it possible to observe a decrease in the intensity of the IrIII in favor of the IrIV following the increase of the potential of the anode.
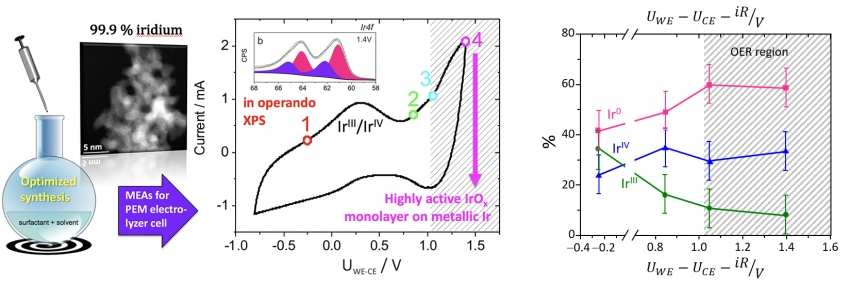
Figure 2: An optimized synthesis lead to high purity nanoparticles. The OER activity of these nanoparticles deposited in a Proton Exchange Membrane was measured using the Near ambient pressure XPS of Sorbonne University on the beamline TEMPO in 10 mbar of vapor pressure. The intensity variations of the different components could be followed according to the applied potential.
These measurements reveal that the nano-catalysts are formed of a metal core surrounded by a thin IrIII/IrIV surface layer of hydroxide and oxide which is progressively transformed into a porous oxide monolayer (IrIV) estimated of about 0.2 nm during the reaction. This transition had already been suggested by electrochemical measurements but never before observed.
Finally, the very high activity of the synthesized nanoparticles can be attributed to their nano-porous structure leading to the formation of a thin oxide surface layer during the OER activity and its interaction with the metal core.
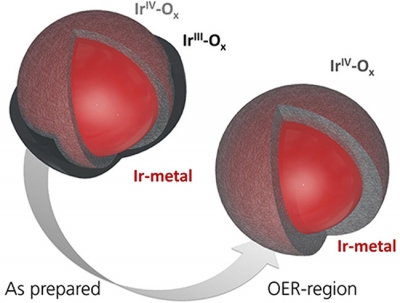
Figure 3: Scheme illustrating the surface change of the nanoparticles structure during the OER.
These experiments also show the interest of operando measurements in photoemission allowing a very high sensitivity to the surface allowing better understanding of the phenomena occurring at the electrochemical interfaces and consequently to guide research on the development of new materials for storage and energy conversion.