A large part of the hundreds of millions of tons of plastic produced each year ends up in nature where it accumulates. Exposed to environmental physicochemical conditions, it fragments into billions of microplastic (< 5mm) and nanoplastic (< 1 µm) debris, impossible to collect and eliminate. This is why scientists are trying to identify organisms capable of biodegrading them.
The GME team of the MICALIS Institute (INRAE/AgroParisTech), the UMR 800 (CNRS/Institute of Physical Chemistry, University of Paris-Saclay) and the SMIS beamline at SOLEIL have investigated whether the Galleria mellonella larva can recycle polyethylene (PE) into biomass, by developing an innovative technique: infrared hyperspectral imaging on tissues of larvae fed with deuterium-labeled polyethylene.
After microorganisms, new targets in the search for candidates to biodegrade plastics are insect larvae, which have shown the ability to chew, ingest and excrete several plastics. Researchers have recently shown that the Galleria mellonella (Gm) larva, or Greater Wax Moth, can chew and possibly biodegrade polyethylene (PE), i.e., break it down into basic molecules, which can be returned to the biological cycle.
The most produced plastic in the world, in the form of high and ultra-high-density PE (HDPE, UHDPE), or low-density PE (LDPE), PE is used to manufacture single use objects (plastic bags, bottles...) but also medical implants. Once accumulated in nature it would take centuries to degrade, some forms being even considered as non-biodegradable. An insect capable of biodegrading PE would therefore be a precious tool for depollution, particularly for eliminating micro- and nanoplastics.
However, although the Gm larvae chew the PE (Figure 1A), it has not been formally demonstrated that they transform it into biomass. Indeed, the scientific literature is ambiguous about the role of the larvae and/or their gut microbiota.
In order to resolve this ambiguity, the research team developed a technique combining infrared hyperspectral imaging of larval tissues and isotopic labelling of PE. Infrared spectroscopy does not allow to distinguish the PE, of chemical formula [-CH2-]n, from the lipids of the adipose tissues of the Gm larva, which are also composed mainly of CH2 (figure 2). Gm larvae were thus fed with HDPE labeled with a tracer: deuterium, a hydrogen isotope. In this labelled HDPE, the -CH2- are replaced by deuterated CD2 groups. But, in infrared spectroscopy, these CD2 groups are strongly distinguished from the CH2 groups of the larval adipose tissue (Figure 2). The normal (CH2) or deuterated (CD2) PEs nevertheless have the same physicochemical properties.
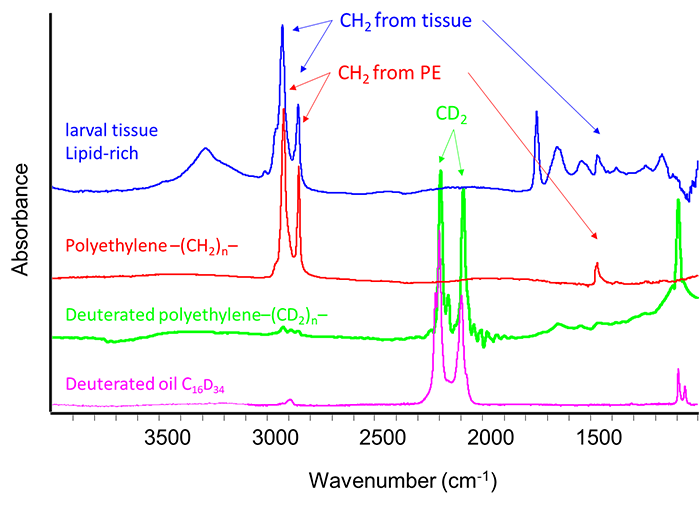
Figure 2. Infrared spectra of fat-rich larval tissue (blue) and PE (red): their CH2 peaks cannot be differentiated. Spectra of deuterated PE (green) and deuterated oil (magenta): the CD2 absorption peaks are easily differentiated from the CH2 peaks of the tissue.
Larvae were sectioned into 10-micron thick sections for analysis by infrared hyperspectral imaging, which allows the reconstruction of chemical maps (Figure 1D).
An initial study showed that deuterium present at natural concentrations in the larvae was undetectable by infrared spectroscopy. After ingestion of 1-4 mg of deuterated oil (C16D34), deuterium becomes detectable in some larval adipose tissue after 24 h and then in all larval tissue after 72 h. Infrared spectroscopy thus allows to detect the bioassimilation of a few milligrams of deuterated food by the larvae and then the integration of the -CD2- chemical groups in their adipose tissues. Moreover, the method demonstrates that the CD2 chains detected in the tissues have a different length than those in the deuterated oil: they have therefore been fragmented and the resulting molecules used to synthesize new biological molecules. The metabolism of the oil is thus proven.
But if the larvae are fed with 2 to 5 mg of deuterated PE, no C-D binding is detected in their tissues. Microscopic particles of deuterated PE are detected throughout their digestive tract (Figure 3) but there does not appear to be any bioassimilation. On the other hand, oxidation of PE and deuterated PE -the first step of biodegradation- was observed in digestive tracts extracted from larvae (ex vivo), probably catalyzed by the intestinal microbiota.
The study also showed that PE alone did not promote larval growth and did not appear to have any nutritional value.
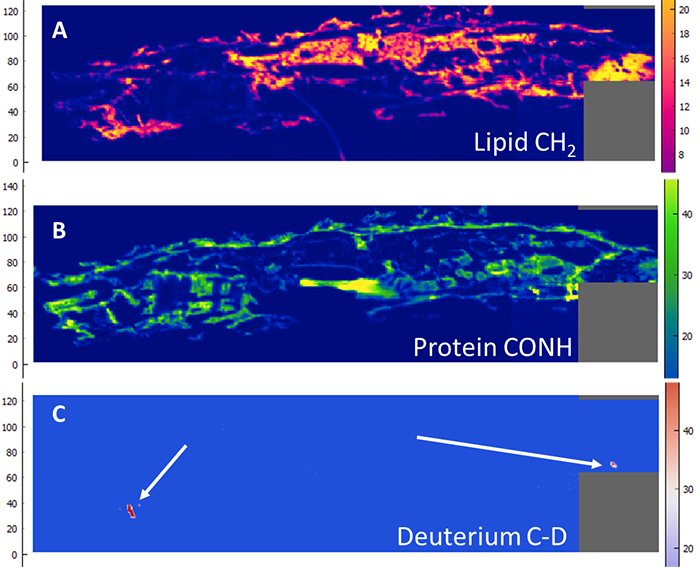
Figure 3. Infrared hyperspectral thin section images of a larva after ingesting deuterated PE. Distribution of A) lipids, B) proteins and C) deuterium (C-D bonds). No deuterium is detected in the tissue but deuterated PE particles are detected in the mouth and rectum of the larva.
In conclusion, this study did not show any bioassimilation of PE by Gm, but its fragmentation into microparticles. On the other hand, the study confirms the hypothesis of the involvement of the microbiota in the biodegradation of plastics as already shown for other insect larvae. The perspectives of this study are furthering the analysis of the roles of the larval microbiota and of the density of the PE.