Hydrogen (H2), as an indispensable clean energy vector, can be produced by biomass decomposition powered by solar light (photoreforming). But a high decomposition extent is required to maximize H2 production per mass of biomass. However, the H2 yield is limited due to incomplete cleavage of the bonds between the carbon atoms of the long sugar molecules (carbohydrates) composing the biomass.
Here, a strategy is proposed to convert carbohydrates into short molecules with only one carbon atom -which are in liquid form, appropriate for transportation- over photocatalyst Ta-CeO2, and is demonstrated in a solar-light-driven flow apparatus. Those carbon molecules can release H2 on-site where needed by photo- or thermocatalysis. This work provides a new perspective for H2 production by photocatalysis.
H2 has emerged as a sustainable and indispensable energy vector that can be produced from renewable biomass with solar energy under mild conditions. However, the intermittent feature of solar light limits the continuous and stable photocatalytic H2 production from biomass. In addition, biomass molecules have robust chemical bonds and various functional groups, which usually result in many side reactions and incomplete cleavage of chemical bonds, especially C−C bond, during photoreforming. These factors limit H2 yield, thereof large-scale biomass utilization. Therefore, preferentially breaking the C−C bond of biomass is expected to deliver a high H2 yield for the purpose of decreasing life cycle carbon emission of photocatalytic H2 production from biomass.
Ta-CeO2 photocatalyst was used for biomass decomposition and was prepared by a solvothermal method (the compounds are synthesized under conditions similar to those in a pressure-cooker). The HAADF-STEM (High-angle annular dark-field imaging-scanning transmission electron microscope) image and the elemental mappings by energy-dispersive X-ray spectra (EDS) of the photocatalyst show the uniform dispersion of Ta and Ce elements, implying that Ta is homogeneously incorporated into the matrix of CeO2 (figure 1).
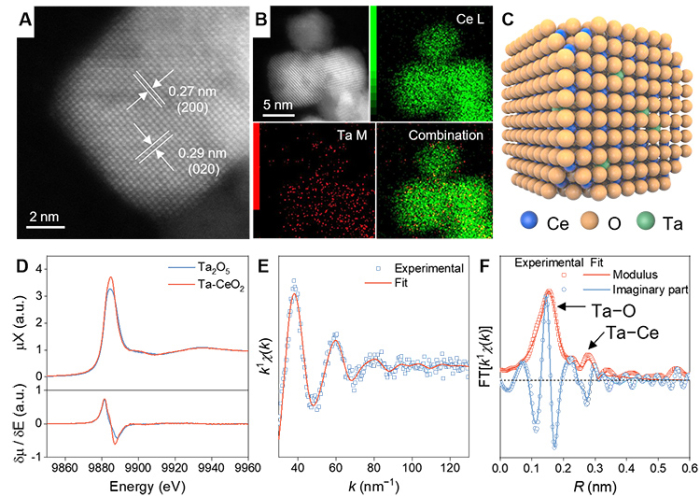
Figure 1: Characterization and structure of Ta-CeO2 photocatalyst:
(A) Representative HAADF-STEM image of Ta-CeO2 nanoparticles;
(B) Representative HAADF-STEM image and EDS mappings recorded at Ce L and Ta M edges -in order to map the 2 elements- and the combination;
(C) Crystal structure of Ta-CeO2 built by referring to the HAADF-STEM results;
(D) XANES spectrum of Ta-CeO2 at the Ta L3 edge compared with that of the standard Ta2O5 ;
(E) fitting of the k1-weighted EXAFS signal at the Ta L3 edge and
(F) the correspondent Fourier transform.
To obtain more structure information on Ta-CeO2, X-ray absorption spectroscopy (XAS) experiments at SAMBA beamline were performed. The inclusion of Ta in the CeO2 matrix is confirmed by X-ray absorption fine structure (XAFS) analysis of the Ta-CeO2 sample (Figure 1 D, E, F). The X-ray absorption near edge structure (XANES) spectrum of the Ta-CeO2 shows that Ta is in the +5 oxidation state as in Ta2O5 (tantalum pentoxide) (figure 1D).
A complementary X-ray absorption fine structure (EXAFS) signal analysis gives access to information allowing the reconstruction of the average local structure around Ta atoms (figure 1E and 1F). The doping Ta5+ partially replaces Ce4+ in CeO2, causing CeO2 lattice distortion and increasing the concentration of Ce3+, which could adsorb more reactant intermediates, thereby breaking the C–C bond more efficiently. The band gap of Ta-CeO2 is narrowing owing to the lattice distortion and increased Ce3+ concentration, resulting in the improvement of the response to visible light.
With the assistance of heating and under the irradiation of visible light, Ta-CeO2 can catalyze the C−C bond cleavage of various sugars, bio-polyols and native biomass to C1 molecules (1 C atom) in yields ranging from 62% to 86%. Heating suppresses unfavorable radical coupling side reactions during photocatalytic polyols oxidation, and the obtained formic acid HCOOH and formaldehyde HCHO molecules are excellent C1 liquid hydrogen carriers (LHCs), which are stable, appropriate for transportation, and can be fully decomposed to release H2 by photocatalysis or thermocatalysis.
The aqueous solution of LHCs obtained from photocatalytic glucose oxidation was directly used for photocatalytic H2 generation after filtrating the Ta-CeO2 photocatalyst, with 33% yield of H2 which is 2.5 times that of direct photoreforming from glucose (figure 2 A&B).
Finally, a lab-scale flow-type apparatus was established (figure 2C) to demonstrate the feasibility of solely utilizing solar energy to provide the thermal and photoenergy to power glucose oxidation to C1 LHCs. Under solar irradiation, glucose was converted to HCOOH and HCHO with formation rates of 2.2 and 0.3 mmol h−1, respectively (figure 2D). After 3-day solar light irradiation with a cumulative irradiation time of 15.5 h, the yield of LHCs reached 15%.
This work provides a new perspective for H2 production and storage by emphasizing the significance of the prioritized scission of biomass C−C bonds to liquid C1 hydrogen carriers.
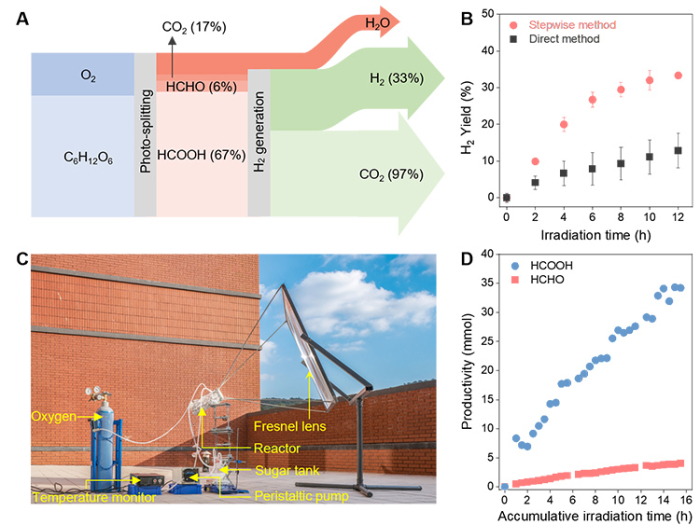
Figure 2: Photocatalytic glucose decomposition to H2 and CO2 and solar-light-driven glucose oxidation to C1 LHCs:
(A) Mass flow of the photocatalytic glucose decomposition to H2 and CO2. The values in parentheses represent the yields of products.
(B) Stepwise photoassisted decomposition of glucose to H2 versus direct photoreforming of glucose.
(C) Photograph of the flow reaction apparatus equipped with a unit focusing solar light.
(D) Reaction results of flow-type glucose oxidation driven by concentrated solar light. The reaction ran for a total of 15.5 h over 3 days.