Although water (H2O) and ammonia (NH3) are very simple molecules, scientific studies on these compounds are still revealing surprising phenomena.
Unlike water, ammonia is not naturally present on earth in large quantities. It has many applications, however, especially in the manufacture of fertilizer, which is why nowadays it is synthesized on a large scale (about 140 million tons/year). The industrial method used for synthesizing it was developed by Haber and Bosch early in the 20th Century (for which they received the Nobel Prize in Chemistry in 1918 and 1931, respectively) and uses the chemical reaction between nitrogen gas N2 and hydrogen gas (H2): N2 + 3H2 -> 2NH3. Historically, this was the first synthesis carried out under high pressure because increasing the pressure of the mixture by several hundred bar tips the chemical balance in the direction of the formation of ammonia. Today, English and French scientists have shown that at pressures of the order of one million times the atmospheric pressure (i.e. 10,000 times greater than that used in the synthesis of ammonia), ammonia becomes unstable and is transformed into an ionic compound consisting of only NH4+ and NH2- ions.
The solid "ice" forms of water and ammonia are naturally present in the Universe and are the principal constituents of the central layers of the Jovian planets such as Neptune and Uranus. This ice comes in many shapes and its properties are closely related to pressure and temperature conditions. It was recently shown that, at high pressure and high temperature, these ice forms become "superionic" (see Figure 1a), a condition in which protons can move almost freely through the fixed network of "heavy" ions (oxygen for water and nitrogen for ammonia): these ice phases have characteristics of both a liquid and a solid.
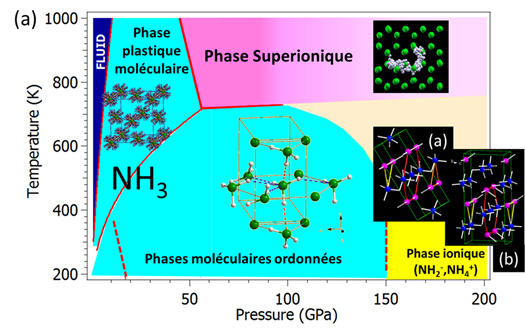
Figure 1 (a) : Ammonia phase diagram. Molecular phases (in which the ammonia molecule NH3 is stable) are shown in blue. Among these phases, there are plastic phases (in which the molecules can rotate almost freely) and ordered phases. At high pressure, the molecular nature of ammonia disappears, giving way to an ionic or superionic solid, depending on the temperature. In the illustration of superionic ammonia (top), following computer simulations, we can see the distribution over time of a single proton (white spheres) through the fixed nitrogen network (green spheres). At lower temperatures, ammonia undergoes self-ionization and adopts one of two ionic structures shown below (the nitrogen atoms of the NH4+ and NH2- ions are represented by blue or pink spheres, respectively).
At room temperature and under moderate pressure, the ice forms of both water and ammonia are molecular solids in which strong covalent bonds and weak hydrogen bonds coexist. In water ice, the effect of high pressure is to increase the hydrogen bond to the detriment of the covalent bond in order to obtain, at 60 GPa, a symmetric ice in which the proton is situated halfway between the two neighboring oxygen atoms. This symmetric state has long been "fantasized" and sought after in ammonia ice up to 200 Gpa, but without success. Recently, ab-initio calculations predicted that instead of becoming symmetric, a proton transfer occurs between the molecules, resulting in an ionic form of ammonia consisting of NH4+ and NH2- ions. By combining infrared spectroscopy, Raman spectroscopy and X-ray diffraction experiments, scientists from the Institute of Mineralogy, Materials Physics and Cosmochemistry (IMPMC) and their collaborators have shown experimentally for the first time this ionic state of ice by applying pressures over 150 GPa. The infrared absorption spectra measured under high pressure (Figure 1 (b)), obtained on the SMIS beamline at SOLEIL, clearly show the molecular-ionic nature of the phase transition. The appearance of strong absorption spectra between 2300-2700 cm-1, which is absent in the molecular phase, is the signature pattern of NH4+ ion elongation (Figure 1b). To determine the crystal structure of this ionic solid, X-ray diffraction imaging was carried out on the ID 27 beamline at ESRF. In parallel, new theoretical calculations were carried out in collaboration with the British research group to investigate possible stable structures. All the experimental data have been interpreted as showing the combined presence of two structurally very similar ionic structures that are theoretically predicted to be stable in this pressure range.
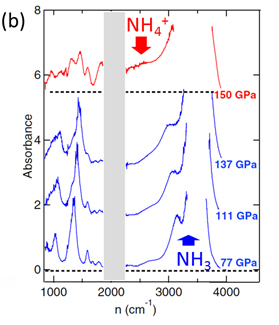
Figure 1(b) This ionization has been shown experimentally by the sudden appearance of the vibrational spectrum of free NH4+ ions on the infrared absorption spectra above 150 Gpa.
This ionization, obtained only by applying high pressures, is a surprising phenomenon that has no equivalent at ambient pressure. Such a state has never been observed in water ice, but could exist, according to recent calculations, in ammonia/water mixtures that make up the interior of giant planets. Indeed, a phase consisting of NH4+ and OH- ions and OH would be stable above 10 GPa in a 50/50 blend, or ten times lower than the pressure conditions for pure ammonia. On this basis, IMPMC scientists are continuing their investigations.