X-ray radiography based on absorption contrast is one of the efficient techniques allowing probing matter in depth. However, its analysis capabilities are strongly limited, when the elementary parts of the sample under test have similar absorption properties. As in microscopic imaging, one way to overcome this limitation lies no more in looking at the absorption-related variations, but at the phase shifts introduced by the materials being passed through: this is commonly known as phase imaging.
In X-rays, this requires an extremely high precision and sensitivity on the phase measurements, since the refraction indices are very close (slightly less than 1, by few factors of 10-6). X-ray interferometry, based on the use of transmission gratings, is one of the techniques allowing to achieve the required performances, with micronic to sub-micronic spatial resolutions on sample. Such a device has been developed within a collaboration between the SOLEIL Metrology and Tests beamline and the “Département d’Optique Théorique et Appliquée” (DOTA) of the French Aerospace Laboratory ONERA located at Palaiseau.
Quadriwave lateral shearing interferometry under broadband illumination: a new achromatic and continuously self-imaging operating regime
The quadriwave lateral shearing interferometer is based on a two-dimensional and binary (0, p) phase grating allowing to generate several replicas of the incoming wave, slightly shifted laterally. Under monochromatic illumination, the interferences between these replicas produce periodically, during light propagation, an image of the diffractive plane. This phenomenon (Talbot effect) appears at discrete distances (fractional Talbot planes), which depend solely on the working wavelength and the pitch of the phase grating. Most grating interferometers developed for X-ray applications are based on this principle. However, they suffer from their chromatic property and need to be resized according to their wavelength of use. The originality of the interferometric bench developed at SOLEIL lies in the use of the panchromatic Talbot effect; an operating regime allowing generation of a propagation-invariant fringe pattern, as soon as the illumination spectral bandwidth is sufficiently large [1]. This achromatic and continuously self-imaging regime was demonstrated experimentally, for the first time in X-rays, on the Metrology and Tests beamline using the white radiation of the synchrotron source [2] – it gives the device a continuously adjustable sensitivity, depending only on the distance between the phase grating and the fringes observation plane.
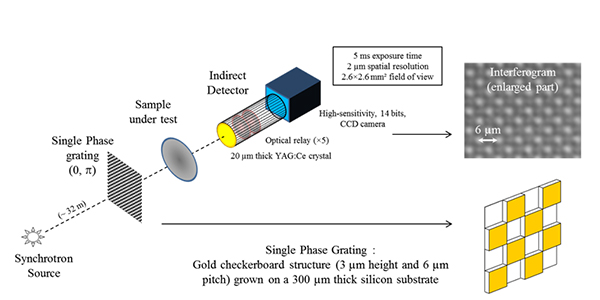
Figure 1: Principle of the quadriwave lateral shearing interferometer, with its binary (0, p) checkerboard phase grating and the generated interferogram (cartesian fringe pattern) obtained on the white beam of the synchrotron SOLEIL Metrology and Tests Beamline.
A single interferogram allows access to the phase gradients in both dimensions, which satisfies the requirement for a robust reconstruction of the wavefront. The phase variations introduced by any object placed on the beam path induce a deformation of the interferogram, from which one can access to the phase gradients of the object.
Two major issues for quantitative phase imaging: absolute calibration and evaluation of the measurement error
Although a qualitative diagnosis may be sufficient in certain cases, many critical applications require the observed phase variations to be precisely quantified. This is particularly the case in the field of X-ray optic metrology, which is essential for the optimization of sources, X-ray optical devices and beamlines. But the need arises as well in biology, in the medical sector, or for non-destructive inspection and testing of materials.
Considering the short wavelengths of the X-ray domain, the optical path differences to be analyzed may be as small as a picometer (a billionth of a millimeter). The access to high-precision absolute quantitative measurements (typically accurate to one tenth of a picometer) implies the use of very high-quality and perfectly characterized optical components and detectors, the development of appropriate calibration procedures, as well as the optimization of algorithms together with the development of new mathematical methods for image processing, data analysis and the evaluation of the measurement error.
In this context, works aiming in the absolute calibration and the deep characterization of the interferometric bench have been conducted, in order to demonstrate that its measurement performances could join those obtained with Hartmann wavefront sensors, introduced in X-rays in the early 2000s. However, the approach applied here consists in using a reference sample in transmission instead of a diffraction-limited reference wave, which is not always easy to create with X-rays on sufficiently large fields. The reference sample used is composed by two perpendicular bevels (150 µm wide) obtained by chemical etching of a 300 µm thick silicon membrane. The chemical etching, which was performed along the crystal (111) reticular planes, gives this prismatic structure a perfectly known and controlled thickness variation (Figure 2a). Thus, it was demonstrated that it was possible to calibrate the experimental setup precisely by use of a high-quality canonical defect. The optical path difference (or phase shift) introduced by the sample was measured after calibration of the device at 210 pm Peak-to-Valley, which is consistent with the etching depth (211 µm) and the refractive index of silicon.
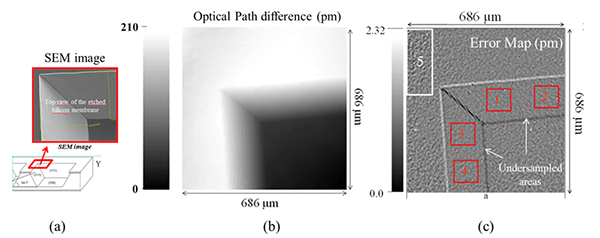
Figure 2: (a) Sketch and SEM image of the reference sample, (b) X-ray optical path difference and (c) error map, measured on the Metrology and Tests beamline.
Simultaneously, theoretical works have been conducted by the Metrology beamline and ONERA teams to go forward and demonstrate the possibility to evaluate precisely the measurement error from the measurement itself. This is a much better approach than the one consisting in considering the measurement error evaluated from a known reference sample (or calibration standard) as generally applicable to any kind of sample. Indeed, it is easy to show that the error on the phase measurement depends on the complexity of the phase to be measured.
Phase derivative closure maps were established: they demonstrate subpicometric accuracy on the phase measurement and reveal not only the measurement noise level, but also the under-sampled areas of the object, phase dislocations, as well as any defect of calibration, device or experimental setup (Figure 2c). In the four areas (1, 2, 3 and 4) of the bevels, the measurement error was estimated at 0.7 pm RMS. Under-sampling defects at the edges of the etched structure are clearly visible and lead locally to higher phase errors. A higher noise level can also be observed in area 5 (top left corner of the image); it comes from a slight defocus of the imaging system, which occurred during acquisition of the interferogram. It highlights the importance of maintaining perfect control over the thermo-mechanical stability of the bench, especially critical on synchrotron radiation given the high powers that can be delivered under white beam operation.
Figure 3 (below) shows a similar analysis performed on a fossil specimen: a mosquito trapped in amber. The measurement error was estimated at 0.08 pm RMS in the homogeneous parts of the abdomen (area A). Larger errors are observable when the frequency of the phase variations introduced by the sample exceeds the sampling frequency of the phase grating, or when the phase contrast introduced by the sample alters the contrast of the fringes in the detection plane.
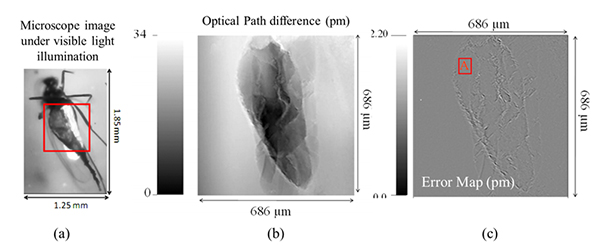
Figure 3: Mosquito trapped in amber – (a) visible microscopy image, (b) X-ray optical path difference and (c) error map, measured on the Metrology and Tests beamline.
These studies are contributions to the field of quantitative X-ray phase imaging. The establishment of such relevant two-dimensional phase error maps is crucial not only for X-ray optic metrology, but also for absolute and quantitative X-ray phase imaging and X-ray phase tomography.
Potential and Prospects
The use of the panchromatic Talbot effect on synchrotron radiation is one of the main original features of this interferometric device, which aims to be as simple as possible to use. But it has also been demonstrated that, under short-wavelength polychromatic illumination, the combination of a phase grating with a pure amplitude grating, ideally required to obtain this operating regime, can be replaced by a single phase grating, which is obviously an advantage in terms of alignment, stability and robustness. Moreover, the phase grating is an optical component with a low aspect ratio, much easier to realize than a pure amplitude grating, relaxing again a number of technological constraints. Finally, it is compatible with illuminations of high numerical aperture, which will allow to improve the spatial resolution on sample. These characteristics naturally open the way to the development of a monolithic apparatus, whose absolute calibration could be maintained permanently, and easily transposable onto highly divergent beams. Using a broadband spectrum (white beam) also offers additional potential. The high photon flux, thus accessible, allows measurement times to be significantly shortened. Using highly sensitive and fast frame rate cameras, a phase measurement could be performed in a few milliseconds under these conditions, on millimetric fields of view with micronic spatial resolutions; tomographic data collection for a three-dimensional rendering of the object in a few minutes only. The constraints concerning the stability of the beam and the experimental setup are thereby also strongly relaxed. Finally, this development opens the path to many applications using commercially available “table-top” X-ray sources, which are widely used in research laboratories, the medical sector and industry.
Two interferometric benches are currently under development. The first one will be optimized for optic metrology at the diffraction limit and macroscopic phase imaging on low-divergence or collimated beams. The second one will be dedicated to high-resolution X-ray phase imaging using illumination of high numerical aperture. The latter one should allow to reach submicronic spatial resolutions on sample, and will be the first step towards the transposition to laboratory X-ray sources. The need lies in several applications of synchrotron radiation, but it is also planned to meet non-synchrotron requirements in a variety of research fields such as biology, medical imaging, materials analysis and microelectronics in particular.
This development work received support from the RTRA « Triangle de la physique » in the frame of the « Mosquito » project. The work currently in progress is funded by the Labex-PALM in the frame of the « NanoXDiag » project.
References:
[1] N. Guérineau et al, “Talbot experiment re-examined: demonstration of an achromatic and continuous self-imaging regime”, Opt. Com., 180, 199–203 (2000).
[2] J. Rizzi et al, “Quadriwave lateral shearing interferometry in an achromatic and continuously self-imaging regime for future X-ray phase imaging”, Opt. Lett., 36(8), 1398–1400 (2011).