Many synchrotron experiments using X-ray fluorescence techniques are currently limited by the sensitivity of dedicated detectors. Indeed, in this type of experiments, key parameters for the detector are their ability to count a high number of incoming photons without saturation and the quality of the signal detected compared to the background noise. For the development of a new generation of fluorescence detectors, a new simulation chain has been built by the Detector group to study the detector performance. Experimental data from the SAMBA beamline has been used to set-up the simulation chain.
X-ray fluorescence (XRF) techniques use multi-element semi-conductor detectors (like Silicon Drift Detectors or germanium detectors) to identify and quantify the chemical element composition of a sample. The detector sensitivity is determined by three main performance features:
- the maximum counting rate, which determines the maximum intensity of fluorescence lines emitted by chemical elements.
- the energy resolution, i.e., how resolved are the fluorescence lines.
- the signal-to-background (S/B) ratio, i.e., the ratio between the fluorescence line intensity and the background - coming essentially from the Compton level.
The two last features are highly affected by an effect called ‘charge sharing’ events, i.e., events where photon energy is shared between neighbouring pixels. This undesirable effect causes an intensity reduction of the main fluorescence signal, an increase of the background level, resulting in a degradation of the energy resolution. The source of these charge sharing events are split events, where charge carriers created by impinging photons at the detector frontside spread over multiple neighbouring pixels when drifting to backside pixels. These events are usually removed in current commercial detectors using a collimator/mask in front of the germanium crystal, at a cost of a significant reduction of the detector active area. This technical solution is undesirable in detectors where smaller pixel sizes will be required.
In this work, a first complete and fully operational simulation chain based on Allpix Squared framework has been built by the Detector group, customized to multi-element germanium detectors, and combined with three-dimensional simulations of the electric field and the weighting potential, based on COMSOL Multiphysics® software. It is worth mentioning that Allpix Squared was originally developed for silicon detectors in High Energy Physics experiments at CERN. A commercial detector, currently used at SAMBA beamline, has been taken as a detector model (see Figure 1) and its performance to direct X-ray beam has been simulated with and without collimator.
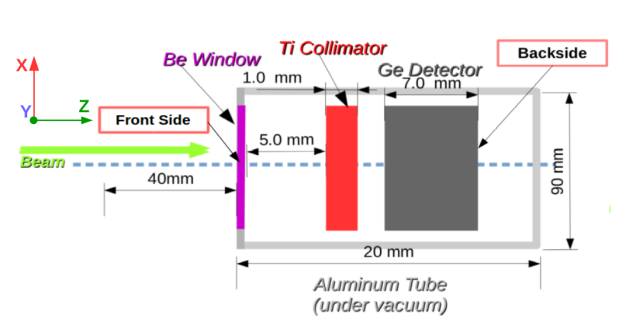
Figure 1: Schematic representation of the multi-element germanium detector model showing its different components. The frontside is the side facing the incident X-rays.
As expected, first results obtained in simulation (see Figure 2) show a reduction of charge sharing events and an increase of the S/B ratio for X-rays with energies below 30 keV if the detector is equipped with a collimator, while small difference between both detector configurations is observed at higher energy. We must note that the detection area is increased by 30% in absence of collimator which represents a significant improvement for future experiments if we are able to deal with charge shared events.
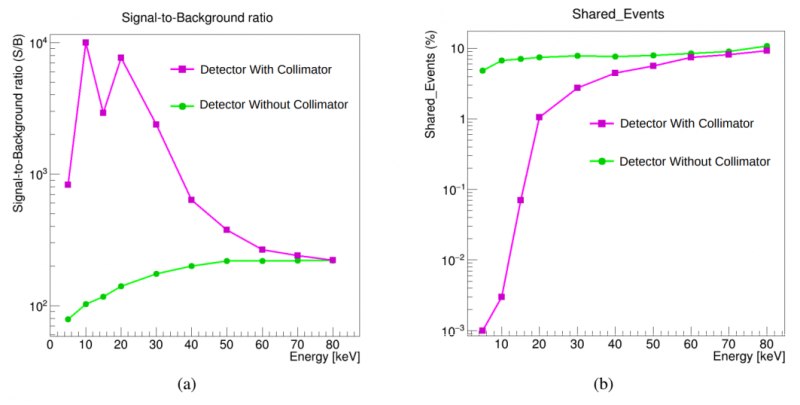
Figure 2: The signal-to-background (S/B) ratio
(a) and the number of charge sharing events
(b) as a function of the incident photon beam energy for a multi-element germanium detector with and without collimator
Experimental data taken at the SAMBA beamline in 2020 and 2021 has been used also to setup and validate the simulation chain. In the XRF experiment performed, a beam with an energy of 28 keV was focused on a reference sample rich in cadmium, situated at 45 deg between the beam and the detector. The comparison of measured and simulated energy spectra (see Figure 3) shows a fair agreement, especially for the main fluorescence lines (cadmium, iron, etc). Some differences have been observed at the Compton level created by the cadmium line and the presence of the titanium fluorescence lines from the collimator, and they will be corrected in the future with a more precise detector modelling.
Our simulation chain provides a powerful tool for the development of semiconductor detectors in general, and in particular for a new generation of germanium detectors. Pixels of different sizes, thickness, arrangement, and geometries (not only square pixels) can be simulated. Current and future studies will aim at optimizing the detector design to enhance its sensitivity for detecting chemical species with very low concentrations in a reasonable time.
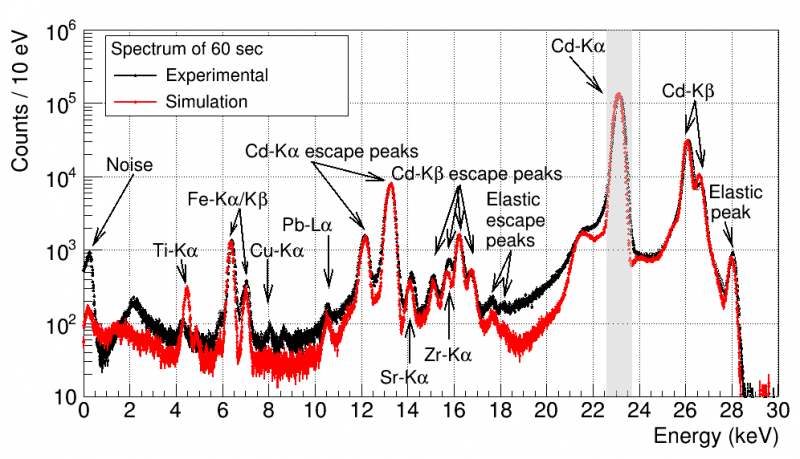
Figure 3: Simulated and measured energy spectrum from a reference sample rich in cadmium measured at the SAMBA beamline.