The use of hydrogen H2 as a clean energy carrier is one of today's technologically viable avenues1. Hydrogen can be produced by water photoelectrolysis: an electrolysis reaction using electrodes activated by sunlight. Hematite, one of the most abundant materials on Earth, is a photosensitive material that can be used to manufacture anodes for this type of reaction.
Using simple methods such as annealing under a controlled nitrogen atmosphere, scientists from SPEC, HERMES beamline and ICPMS have succeeded in improving hydrogen production by hematite-based photoanodes. State-of-the-art instruments operated at the HERMES beamline allow understanding the origin of this improvement, and pave the way for simple, affordable and effective approaches optimizing materials for tomorrow's photoelectrolysis cells.
Hematite, Fe2O3, can be used to produce hydrogen by dissociating water in a photoelectrolysis reaction. Indeed, part of the energy consumed in an electrolyzer2 can be substituted by direct absorption of solar radiation, using photosensitive anodes such as hematite. The band gap of this semiconducting oxide is 2.1 eV, corresponding to the energy of visible sunlight. When this light is absorbed, an electron-hole pair is generated in the hematite: the electron will reduce the water at the cathode, while the hole will oxidize the water at the anode. The photocurrent generated is proportional to the quantity of H2 molecules produced. In theory, it should reach 12 mA/cm2 at a potential of 1.23 V. In practice, the photocurrent does not exceed few hundreds of µA. The main culprit is the holes mean free path3, which is extremely small in hematite, on the order of 2 to 4 nm. To overcome this obstacle, various electronic band engineering techniques are employed, more or less complex, all aiming at modifying the electronic properties of hematite.
In a joint study, scientists from the Service de Physique sur l'État Condensé (SPEC: UMR CEA - CNRS) at l'Orme des Merisiers, the Institut de Physique et Chimie des Matériaux de Strasbourg (IPCMS: UMR CNRS - Université de Strasbourg) and SOLEIL's HERMES beamline, have determined the origin of the performance enhancement of Ti-doped hematite-based photoanodes, synthesized under a controlled nitrogen atmosphere. The synthesis is carried out in 2 stages: during the first, Ti-doped akaganeite, Fe3+O(OH,Cl) is obtained, in the form of a carpet of nanorods on FTO4 substrates by aqueous chemical growth following a hydrothermal process.
During the 2nd phase, the samples are annealed at 600°C, leading to a phase transformation into hematite. It is the control of the annealing atmosphere (air or nitrogen) during this 2nd stage that enables improving the water splitting performance.
A key feature of this study is the complementary use of the full range of experimental methods available at the HERMES beamline. XPEEM (X-ray photoemission electron microscopy), which detects only electrons coming from the topmost atomic layers of the analyzed material, makes it possible to separate information related to composition and chemical coordination originating from this surface, from that originating from the bulk of the samples. To achieve this, the researchers employed a methodology taking advantage of the specific geometry of the XPEEM microscope: the "shadow XPEEM".
The results show, in the case of Ti-doped hematite annealed in a nitrogen atmosphere, the surface segregation of a Ti-rich phase and the formation of new electronic states corresponding to surface states (Figure 1).
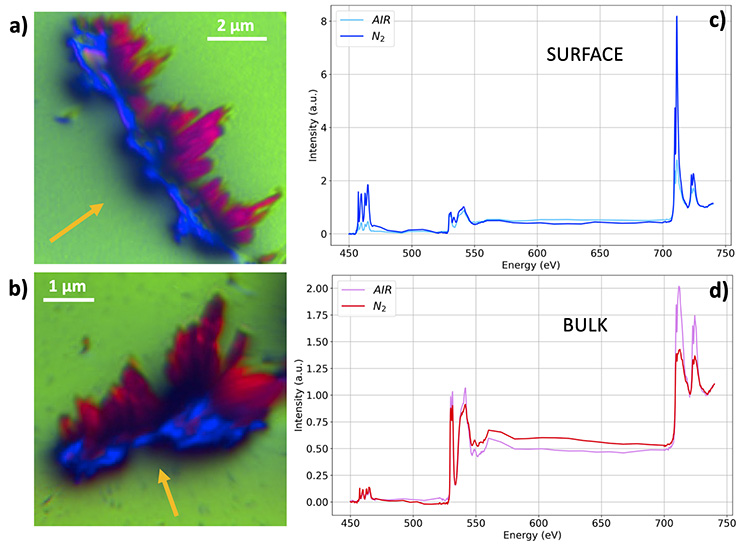
Figure 1: XPEEM results obtained by comparing air-annealed (a) and nitrogen-annealed (b) samples, following the color code: red - bulk signal (shadow), green - gold support background signal, and blue - surface signal. The corresponding X-ray absorption spectra demonstrate the formation of a Ti-enriched phase at the surface of the nitrogen-annealed samples (c), while the volumes show very similar spectra (d).
Scanning transmission X-ray microscopy (STXM) was used to complement XPEEM measurements to better localize this Ti-enriched phase, by probing material thicknesses of a few hundred nanometers. It was possible to isolate regions of a few tens of nanometers whose spectral signature differs from that of Ti-doped hematite, demonstrating the presence of a chemical coordination specific to a pseudo-brookite-type phase (Fe2TiO5 or FeTi2O5).
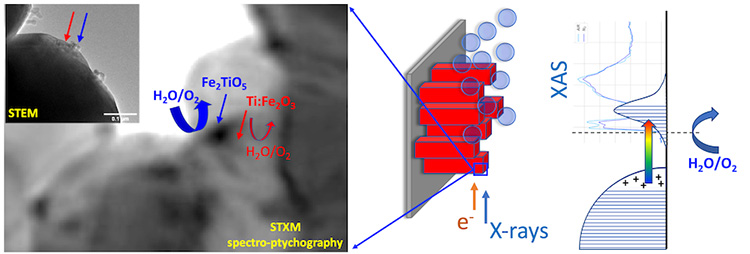
Figure 2: left - image showing STEM/ptychography correlation, highlighting pseudo-brookite clusters (blue arrows) on the surface of a Ti-doped hematite nanorod (red arrows); right - sketch of hematite nanorods morphology and electronic bands (filled and empty states) probed by X-ray absorption.
Spectro-ptychography allowed to improve the spatial resolution and locate pseudo-brookite clusters more precisely. The sensitivity of the approach is remarkable: it enables to differentiate between two Ti-containing phases with an energy shift of just ~0.1 eV for the peak at 457.5 eV (see Movie). This is a direct demonstration of the power of such spectromicroscopic methods, a textbook case of how sensitivity to chemical coordination can improve spatial resolution.
The study shows that controlling the annealing atmosphere, a simple physico-chemical parameter, it is possible to fine-tune the hematite/electrolyte interface at nanometric scale for a net improvement in activity during the water dissociation process.
Movie: Transmission-mode hyperspectral result of spectro-ptychography obtained at the Ti absorption edge (1st part of the film, spectra 456.5-468.5 eV) and O edge (2nd part, spectra 529-535 eV). Thanks to the high spectral sensitivity of this technique, pseudo-brookite clusters appear highly contrasted at their absorption maximum ("surface" part, at 457.5 eV). Image size: 2.5 x 2.5 µm2.
-------------------------------------------------------
[1] - https://www.economie.gouv.fr/industrie-nouvelle-strategie-hydrogene-pour-la-france
2 -An electrolyzer dissociates water using two metal electrodes, the anode and cathode, by applying a potential difference 1.23 V higher than the water's redox level. This generates oxygen at the anode and hydrogen at the cathode.
3 - Mean free path: distance over which a charge can move without undergoing recombination.
4 - FTO: Fluorine-doped tin oxide is the substrate of choice for all solar applications.