Through X-ray absorption and x-ray magnetic circular dichroism measurements, researchers at the Institut Jean Lamour (Université de Lorraine, Nancy), the Paul Scherrer Institute (Villigen, Switzerland) and the ODE beamline (SOLEIL Synchrotron) have, for the first time, identified in a heavy fermion system, changes in the charge state and magnetic moment of ytterbium associated with quantum criticality.
Quantum criticality, which is considered to be behind unconventional superconductivity, could play a role in quantum information processing. Changes in the electronic properties of ytterbium identified in this way persist at room temperature due to the energy levels involved.
Quantum criticality is investigated within various families of materials notably high-temperature superconductors, quantum magnets or heavy fermion systems. A heavy fermion system is an intermetallic alloy where magnetic electrons from the incomplete inner 4f (lanthanide) or 5f (actinide) layers hybridize with conduction electrons to form fermions that behave like substantially heavier particles. When one of these systems exhibits a second-order phase transition, i.e. a continuous transition between the two phases, at a specific temperature known as critical temperature, this can be brought to absolute zero by means of a control parameter (external pressure, chemical composition or magnetic field). The singular point separating the two ground states is called the quantum critical point. Quantum fluctuations in the vicinity of this point induce profound transformations in the electronic properties of solids at finite temperature, this is called quantum criticality.
In addition to the fundamental interest of this work, quantum criticality, which is believed to underlie unconventional superconductivity1, could play a role in quantum information processing.
Most of the heavy fermion intermetallic systems studied so far combine an unstable 4f layer element, such as Ce or Yb, with non-magnetic elements (Al, Si, Ge, Cu, Rh …). Quantum criticality then manifests itself most often at low temperature in the vicinity of the element with 4f magnetic instability. This results from the competition between the Kondo effect2 and the RKKY interaction3, these two interactions depending differently on the hybridization between localized 4f electrons and conduction electrons.
In the YbMn6Ge6-xSnx series, the manganese sublattice is highly magnetic [Fig. 1]. The competition then involves the Mn-Yb interaction, which is much more intense than the usual RKKY interaction, such that ytterbium magnetic instability occurs at strong hybridisation where load fluctuations play a role. It is the higher levels of energy than in standard heavy fermion systems that explain the persistence of quantum criticality at high temperature.
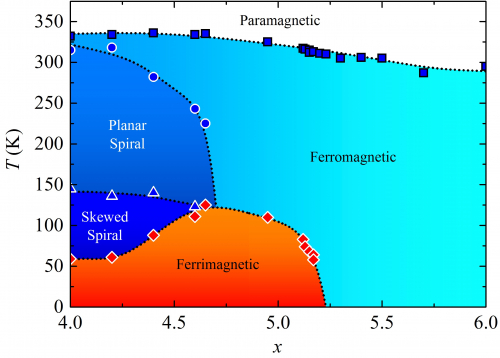
Fig 1: (x, T) magnetic phase diagram of YbMn6Ge6-xSnx (4 ≤ x ≤ 6) system. The regions in blue indicate the composition and temperature domains where the manganese sublattice alone is magnetically ordered. The red area indicates the domain where ytterbium is also ordered. Ytterbium magnetic instability occurs at xc ≈ 5.23.
Quantum criticality is usually revealed through thermodynamic or transport measurements. Electron spectroscopy techniques [X-ray Absorption Spectroscopy (XAS) and X-ray magnetic circular dichroism (XMCD) at the Yb L3 edge] were used here. These measurements revealed a peak in the evolution due to the tin content in the ytterbium valence (extracted from the XAS measurements) and its magnetic moment (linked to the XMCD signal) in the vicinity of the magnetic instability [Fig. 2]. These observations are consistent with recent theoretical predictions in unconventional quantum criticality.
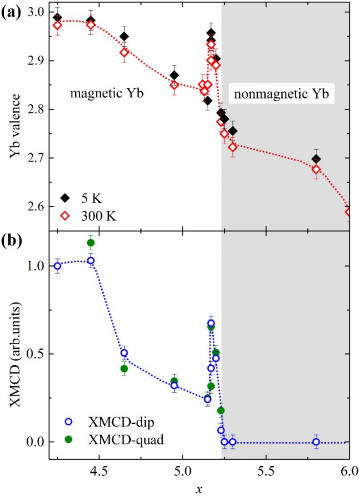
Fig 2: (a) Evolution of ytterbium valence at 300 K and 5 K with the tin content in YbMn6Ge6-xSnx (b) Evolution of dipolar and quadrupolar dichroic signals at 5 K.
The XAS and XMCD measurements were performed on the SOLEIL synchrotron (energy dispersive EXAFS) ODE beamline at between 300 K and 4.2 K in a magnetic field that can reach 1.5 T. The dispersive mode enables the acquisition in a relatively short time of a stable signal due to the absence of mechanical movement.
The work on YbMn6Ge6-xSnx shall be continued and extended with, notably, transport measurements on single crystals and XAS and XMCD measurements under external pressure on the ODE beamline. More generally, these results open new experimental and theoretical research avenues related to quantum criticality in 3d-4f intermetallics.
The project was partly co-financed by Campus France and the Schweizerische Akademie der Technischen Wissenschaften SATW as part of the Germaine de Staël programme.
--------------------------------
1 Discovered in 1913, the superconductivity phenomenon was explained in 1957 by the Americans Bardeen, Cooper and Schrieffer ("BCS" theory): electrons group together in pairs to form "Cooper pairs" that can move without friction, therefore without resistance, like a wave propagating within a solid. This theory explains the behaviour of so-called conventional superconductors, whose critical temperature (where superconductivity is observed) is close to absolute zero. However, there are other superconductor materials, so-called unconventional materials, which often have higher critical temperatures. However, the origin of superconductivity within these materials remains an enigma and is notably not explained by the BCS theory.
2 The Kondo effect refers to the specific low-temperature behaviour of certain electrical conductors. The electrical resistivity of solids such as metals generally decreases with temperature, stabilizing at a constant value. Under certain conditions, the behaviour of these materials can be modified by adding magnetic impurities (dilute magnetic alloys), so that below a temperature TK, which can vary from a few Kelvin to several hundred depending on the alloy, the resistivity of the doped material increases again. The Kondo effect refers to this increase in resistivity at low temperatures. The Kondo effect arises when the magnetic moments are shielded by conduction electrons.
3 RKKY coupling (for Ruderman-Kittel-Kasuva-Yosida) is a coupling interaction mechanism of nuclear magnetic moments or localized electron spins in the inner layer of a metal via conduction electrons.