Metallic glasses are currently of growing interest worldwide due to their remarkable physicochemical properties with regard to their crystalline counterparts. Among them, the cerium based metallic glasses (Ce-MG) distinguish themselves by the existence of polyamorphism under pressure: phase transition between amorphous phases with change of density and local structure at transition. Cerium based metallic glasses have been studied on the ODE beamline in order to better understand this polyamorphism.
Recently, rare earth based metallic glasses have been found to display thermo-plastic behavior with very low glass transition temperature (Tg<373K) and low Young's modulus in comparison to classical amorphous matter [1]. At room temperature, these alloys are strong (elastic strain of 2 % [2]) and brittle. In their supercooled-liquid state, these materials can however be repeatedly shaped into very fine structures down to the nanometer-scale, of great interest for micro electromechanical systems or nanotechnology applications such as high density data storage [3].
Among them, the cerium based metallic glasses (Ce-MG) distinguish themselves by the existence of polyamorphism under pressure (phase transition between amorphous phases with change of density and local structure at transition).
This transition, unexpected in these spatially compact systems, leads to structural changes under pressure which were not clearly identified. Furthermore, the role of the cerium constituent during this transition seems decisive but only few works studied the link between the properties of the pure cerium and the polyamorphism in the Ce-BMG [4,5].
Using energy dispersive XANES measured at the ODE beamline at Ce-L3 edge under high-pressure and high temperature, it was observed (Fig 1) that the polyamorphism in Ce-BMG is directly correlated to the electronic configuration of the 4f electron, with a pressure induced-delocalization [6]. The cerium is thus clearly piloting this amorphous-amorphous transition.
This first conclusion is particularly interesting in view of a previous study by our group on the properties of pure crystalline cerium along the γ⇔α transition, transition, also induced by 4f electronic delocalization under pressure. This work has actually demonstrated the existence of a critical point in the p, T diagram of crystalline cerium at the end of γ⇔α transition line. The main question turns thus to the existence of a critical point in the amorphous-amorphous transition in Ce-BMG?
In a first step, high pressure and high temperature XANES measurements have been carried out at SOLEIL (plus x ray elastic diffusion measurements at ESRF) in the 0-15 GPa, 300-340 K ranges. This work has of course to be complemented by further studies in a more extended pressure and temperature ranges, but in the present one, our study [6] did not reveal a clear occurrence of such a critical point in the range of pressure/temperature probed (Fig 2). This work will encourage further investigations on Ce-based metallic glasses phase diagrams in order to support, or refute, the actual theoretical understanding of polyamorphism.
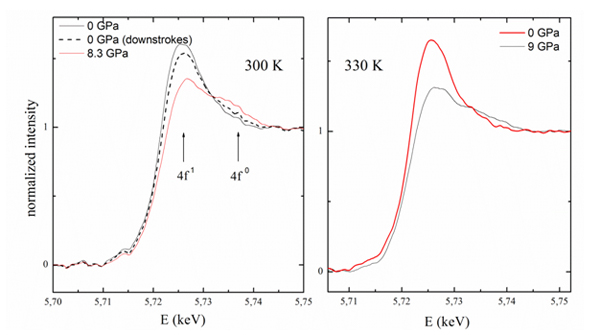
FIG1. Ce60Al20Cu20 metallic glass at 300 K (left) and 330 K (right). Black: ambient. Red: high pressure. Dashed: after decompression. Solid arrows mark the 4f0 (itinerant) and 4f1 (localized) components.
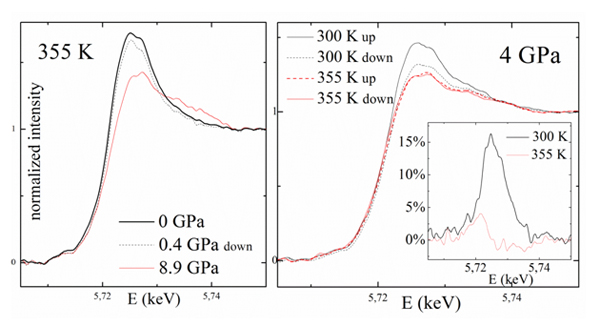
FIG2. Ce69Al10Cu20Co1 metallic glass at 355 K. Black: ambient, red: high pressure, dashed: after decompression. Right: XANES spectra at 300 K (black) and at 355 K (red). Continuous line: 4 GPa upon compression, dashed line: 4 GPa upon decompression. Inset: difference between the XANES at 4 GPa under compression and decompression divided by the compression spectrum (in %).
References:
[1] E. Lutanie, Mater. today 12, 12 (2009).
[2] B. Zhang, D. Q. Zhao, M. X. Pan, W. H. Wang, and A. L. Greer, Phys. Rev. Lett. 94, 205502 (2005)
[3] G. Marsh, Materials Today 6, 38 (2003)
[4] H. W. Sheng, H. Z. Liu, Y. Q. Cheng, J. Wen, P. L. Lee, W. K. Luo, S. D. Shastri, and E. Ma, Nature Mater. 6, 192 (2007)
[5] M. Duarte, P. Bruna, E. Pineda, D. Crespo, G. Garbarino, R. Verbeni, K. Zhao, W. Wang, A. Romero, and J. Serrano, Phys. Rev. B 84, 224116 (2011)
[6] F. Decremps, L. Belhadi, D. Farber, K. Moore, F. Occelli, M. Gauthier, A. Polian, D. Antonangeli, C. Aracne-Ruddle, and B. Amadon, Phys. Rev. Lett. 106, 065701 (2011)