Lighting a candle, a quite ordinary action, starts a highly complex chemical reaction sequence: the combustion of the candle wax. The warm and festive yellow candlelight is radiated from soot particles that are formed from smaller reactive molecules in the flame.
Soot – while it helps to create the cozy candle atmosphere – is one of the most harmful emissions from combustion that affects air quality, climate and human health. Important properties of the emitted soot particles, including their concentration, size, chemical nature and reactivity, depend on the chosen fuel and combustion conditions.
The scale of the emission problem of combustion is enormous and still rising with the number of cars, trucks, planes and power plants worldwide. Indeed, combustion of fossil fuels – coal, oil and natural gas – contributes with more than 80% to global energy consumption today. This huge fraction will decrease only gradually, not least because of the long lifetime of present technology and installations. To make combustion cleaner and to meet tight air quality regulations, it is thus important to understand the chemical reactions that lead to undesired emissions.
Progress in understanding combustion chemistry needs information about many – up to hundreds – of chemical species that can be formed in the combustion reactions. More challenging, some of these species are highly reactive and unstable; they typically occur in tiny concentrations and are hard to quantify. Also, their molecular structure is important for the ways in which they can react. For example, butane gas has two variants or isomers, both with the same chemical formula, but n‑butane, linear and chain-like, and its molecular twin, branched iso-butane, show different flame characteristics and emission potential. Structure identification of all stable and unstable intermediate species formed along the reaction sequence is thus one of the most important steps for improving chemical reaction models. Advanced experiments to discriminate between isomers in flames include synchrotron-based photoionization mass spectrometry, available only at a few facilities worldwide. All species including isomers can, in principle, be ionized selectively with brilliant synchrotron radiation of variable photon energy and detected by the ratio of their mass and charge. Most recently, however, even higher discriminative power has been demonstrated using photoelectron-photoion coincidence (PEPICO) spectroscopy in flames.
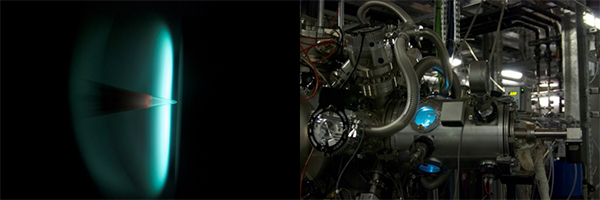
Photo 1: The first flame experiment at the DESIRS beamline using the DELICIOUS3 spectrometer for double imaging photoelectron-photoion coincidence spectroscopy.
a) Flame of the liquid fuel cyclopentene, burnt at reduced pressure with oxygen, to study specific chemical species involved in the early stages of aromatics and soot formation. The chemical species in the flame are sampled through the cone at different positions along the distance from the burner surface through the luminous flame zone and are analyzed with the coincidence spectrometer.
b) Flame chamber mounted to the beamline.
Between the teams of SOLEIL and Bielefeld University, Germany, a new combustion experiment has been set up at the DESIRS beamline, using the DELICIOUS3 i2PEPICO spectrometer to probe the multitude of reactive chemical species in flames with unprecedented photon energy resolution. This experiment, for the first time, has demonstrated the potential of highly multiplexed flame analysis from fixed-photon-energy PEPICO [1]. In a flame of a chemically rather simple gaseous fuel, ethene, it could be shown that isomeric intermediate species can be unambiguously detected from PEPICO measurements using appropriately selected fixed photon energies. Instead of time-consuming scans over large ranges of photon energy to match the ionization thresholds for many important flame molecules, the unique facility available at SOLEIL has enabled to detect relevant stable and radical intermediate species – including twins such as allene and propyne, and triplets such as 1- and 2-butyne and 1,3-butadiene – from a single time-efficient fixed-photon-energy measurement at a given photon energy.
This method is now being extended to using prototypical liquid fuels, including cyclopentene as a conventional hydrocarbon fuel and a small ester as a representative of biofuel combustion chemistry. In both cases, the pathways to form aromatic molecules and soot are different and thus of specific interest. These more complex fuels are closer in nature to the technical fuels in use today, making the results relevant for the critical inspection and further development of combustion models.
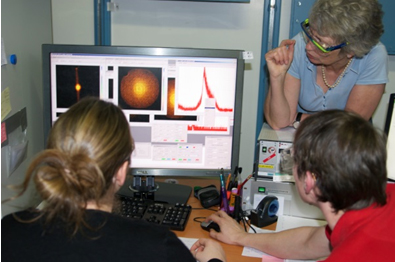
Photo 2: Combustion chemistry followed with the coincidence spectrometer: ion and electron signals and mass spectra provide a first glance into the complex chemistry of a liquid-fuel flame while they are being recorded.
Once such detailed reaction models are validated by high-quality experimental data, they can be expected to simulate the combustion process in more realistic conditions like in an engine to predict emissions and to optimize and control the process.