Experimental endstations are a critical part of a synchrotron facility and should be constructed in perfect coherence with the characteristics of synchrotron radiation so that the users can take full advantage of third generation light sources. One can think of an endstation as the sensor in a camera: for good picture quality, both the lenses (beamline) and the sensor (endstation) must meet the requirements. There is, however, a slight complication, because each user would like to adjust the picture quality to suit his/her needs. Thus, the endstation must be extremely versatile while guaranteeing a certain degree of specialization for tailored individual needs, all in a user-friendly manner and reliably: a true conundrum!
DESIRS, SAPHIRS and DELICIOUS 3
At DESIRS, two permanent endstations are available for external users, one of which, the molecular beam chamber SAPHIRS, is dedicated to valence-shell photoionization processes in the gas phase, during which a neutral molecule is ionized and a cation and an electron are produced. Users and team members alike are interested in a large variety of systems, from bare atoms up to nanoparticles, spanning several scientific fields.
To obtain a complete picture of the photoionization event, scientists start by dramatically reducing the number of initial states available in the neutral through the usage of a molecular beam, or supersonic expansion, where the neutral’s internal energy is converted into kinetic energy along the beam’s direction. Then we study, with as much details as possible, the final state consisting of a departing electron plus cation, and how the initial energy deposited into the system is shared and evolves among the different degrees of freedom (electronic and nuclear continua).
To achieve this goal in the most comprehensive way, the spectrometer, DELICIOUS III, is capable of separating the different photoionization events in time, so that an electron can be correlated to a given ion coming from the same photoionization event, the so-called PhotoElectron PhotoIon COincidence scheme, or PEPICO. More precisely the spectrometer, as shown in Figure 1, is based upon a double imaging concept, which yields the full cation velocity vector, i.e., total kinetic energy and angles of ejection, and correlates this information with the photoelectron velocity mapped images, so that the photoelectrons can be filtered in ion mass, ion direction and ion kinetic energy. For a full description of the performances, the reader is directed to the associated publication cited below.
The multidimensional data delivered by DELICIOUS III can be reduced in many ways—see the N2 example for an overview—and open new and exciting research avenues, notably in photochemistry or clusters science.
Last, but not least, the spectrometer has been conceived in-house at SOLEIL, from the ray-tracing simulations to the mechanical conception, and most of the electronics have been custom-made by nearby groups, perpetuating the know-how within the Orsay/Saclay area.
Example: photoionization of N2 above the first dissociation threshold
The photoionization of N2 at a photon energy of 25.6 eV produces an electron and a N2+ cation with a wide distribution of internal energies, depending on the kinetic energy of the departing photoelectron. If the amount of internal energy is above the dissociation threshold, the parent ion will break to give N + N+. Figure 2 shows the raw coincident photoelectron and photoion images for the N2+ and N+ obtained after applying the mass filter. The position and arrival times of the particles are then extracted and converted to velocity distributions to yield the results displayed in Figure 3.
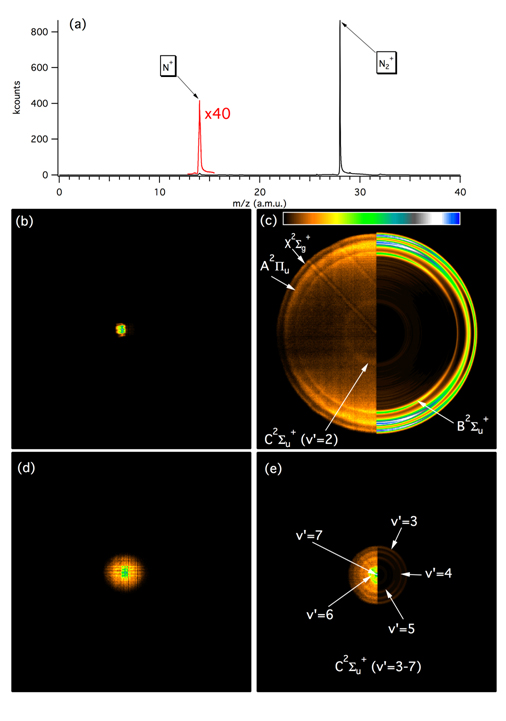
Figure 2: (a) Mass spectrum showing the N2+ and N+ ions. The left figures, (b) and (d) present the photoion images for the N2+ and N+ masses respectively. The right figures (c) and (e), correspond to the photoelectrons correlated to the production of N2+ and N+, resp. In all the 2D images, the radius is proportional to the particles’ kinetic energy. An overview of the information that can be extracted from these images is presented in Figure 3 below.
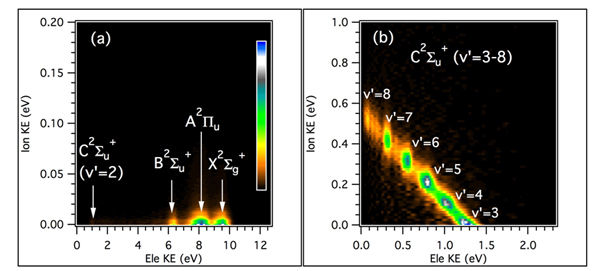
Figure 3: Electron/ion kinetic energy correlation diagrams for the photoionization of molecular nitrogen above the dissociative ionization threshold for the N2+ and N+ masses. When the photoelectron departs, the internal energy of the N2+ ion is given by energy conservation as: hv=Eint + KEele, so that very fast electrons are correlated to ions with small internal energy. Conversely, for slow photoelectrons, the parent ion will have an important energy reservoir that will be dissipated via dissociation to N2+ -> N+ + N. Therefore, panel (a) shows that the N2+ ion has no translational energy (does not come from a fragmentation event), and can exist only for fast photoelectrons. Panel (b) shows that the N+ fragment only appears for slow photoelectrons (KEele<1.25 eV, correlated to the C electronic state of the N2+ cation), and the fact that intensity appears along a diagonal line means that only one dissociation channel is open. The figure provides a very clear picture of the spectroscopy of the parent ion, as well as its fragmentation pathways—appearance energies and barrier heights.