Integration of quantum information hardware into scalable and robust solid-state architectures will be key to allow performing quantum computation, one of the pillars of the recently launched EU Quantum Technologies FLAGSHIP program. Researchers from the Materials Science and Nanoscience Institutes of Aragón in Spain (ICMA and INA, CSIC and University of Zaragoza) and from SOLEIL and the Paris NanoSciences Institute (Sorbonne Université), with the help of the SIRIUS beamline, have developed the means to introduce molecular-based spin quantum bits directly on the surface of devices.
Magnetic molecules with a (pseudo-)-spin-1/2 state represent one of the potential hardware to embody the quantum bit of information, or qubit. Indeed, the two quantized orientations of the electronic spin form a natural two-level quantum system that provides the basis to encode the states of a qubit. This molecular approach towards the elaboration of a quantum computer has the advantage of facilitating the scalability of the qubits. However, the proposed hybrid technology to build a scalable computation architecture based on these molecular qubits requires positioning the molecules at specific localizations of solid-state devices, while keeping a control over their orientation. The authors of the present work argue that such a control of the orientation once deposited on a surface can be enforced by the periodicity of 2D networks in which the molecular qubit would act as node.
To demonstrate the validity of their approach, the scientists have used a Cu(II) porphyrin molecule with four coordinating carboxylic acid groups, [CuTCPP], and formed the targeted solid 2D Metal-Organic Framework (MOF) by reaction with diamagnetic Zn(II) ions (Figure 1, left). The quantum coherence of the [CuTCPP] qubit has been determined through its phase-memory time both isolated and as node in the MOF. The similar values of the order of microsecond indicate that quantum coherence is maintained upon insertion within the 2D framework (Figure 1, right).
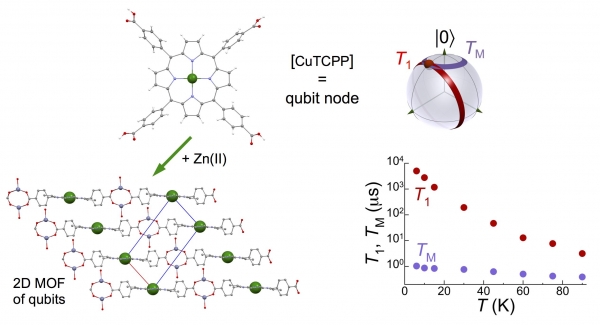
Figure 1:
Left: Structures of the Cu(II) porphyrin molecule [CuTCPP] used as node and of the 2D metal-organic framework formed upon reaction with Zn(II) ions and formation of [Zn2] paddle-wheels through the four carboxylic groups of [CuTCPP].
Right: the Cu(II) spin provides the two-level system able to encode quantum information through the infinity of superpositions of its two states, as typically represented by the points on a Bloch sphere (top, right). Similar spin-lattice (T1) and phase-memory (TM) times have been determined for both the isolated molecule in frozen solution and the 2D MOF (diluted to 1% with [ZnTCPP], bottom right).
The second part of the study shows that nanodomains of the same 2D MOF can be formed at the air-water interface of a Langmuir trough and subsequently transferred as dense films to a variety of substrates. The data obtained by grazing incidence X-ray diffraction at the SOLEIL SIRIUS beamline (Figure 2, right) were key to demonstrate that the same 2D square-grid structure is actually formed in-situ at the interface. EPR spectroscopy then confirmed that the orientation of the [CuTCPP] moieties in the transferred nanodomains is homogeneous and parallel to the substrate.
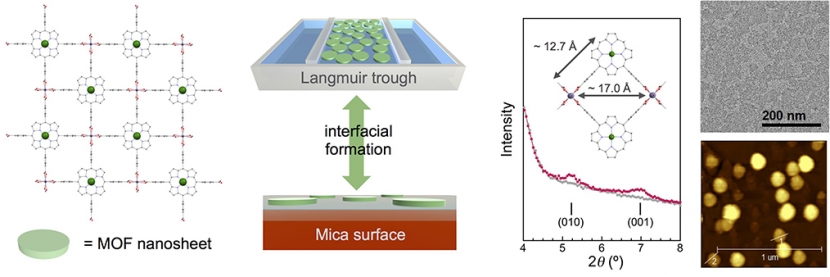
Figure 2:
Left: structure of the square-grid MOF nanosheet similar to those in the bulk solid material that form at the air-water interface either in a Langmuir trough or on Mica.
Right: in-situ synchrotron GIXD data and TEM image for the MOF nanosheets formed in the Langmuir trough and topography AFM image of the same nanosheets formed locally on Mica.
Eventually a protocol for the direct in-situ formation of the domains over a substrate, that mimics the process taking place in the Langmuir trough, was developed. Topographic observations (Figure 2, right) and local chemical analysis confirmed that similar nanosheets of the MOF with qubit nodes are formed at microscopically selected localizations.
Overall, this work demonstrates the potential of a framework approach towards the integration of molecular qubits onto solid surfaces with controlled localization and enforced orientation. Further developments involve the down-sizing of the localization control to the tens of nm range, allowing the coupling of the qubit nanosheets to superconducting resonators resulting in a hybrid quantum processor that will hopefully allow the realization of quantum algorithms.