In the frame of a European Research Council (ERC) Advanced Grant, “COXINEL” (Principal Investigator: M. E. Couprie), a specific manipulation line has been designed and built at Synchrotron SOLEIL. It is now installed at Laboratoire d’Optique Appliquée (LOA), where the LPA electrons are produced and accelerated.
The laser discovery, followed by the FEL (free-electron laser) invention, led to the advent of X-ray FELs opening new areas for matter investigation. In 2018 the Nobel Physics Committee has highlighted the domain of lasers, in giving the award to Gérard Mourou (Professor member of Head-college of École Polytechnique), and to Donna Strickland for their researches on the generation of ultra-short and intense optical pulses, and to Arthur Ashkin for his works on the "optical tweezers" enabling to manipulate living cells, viruses and bacteria.
The invention by G. Mourou and D. Strickland deals with a method of amplification (Chirped Pulsed Amplification) allowing the laser power to be multiplied by a very large factor. Besides the fortuitous discovery of a targeted impact of high power laser on a cornea having led to laser myopia surgery, these ultra-high lasers permit also to create and accelerate electrons up to several GeV on ultra-short distances. The development of high accelerating gradient laser plasma acceleration (LPA) is promising: 100 MeV – 10 GeV energy, kA peak current, ultra-short bunches, 1πmm.mrad normalized emittance beams can be produced. The hopes put in LPA to drive undulator radiation and FEL light sources are challenged by LPA parameters that do not meet conventional accelerator state-of-the-art performance, in particular for the energy spread and for the divergence. Demonstration of a proper electron beam control is the first challenge to overcome in the path towards LPA based FELs and requires specific beam handling.
In the frame of an European Research Council (ERC) Advanced Grant COXINEL (Principal Investigator: M. E. Couprie), a specific manipulation line has been designed (see Fig. 1) and built at Synchrotron SOLEIL considering 200-400 MeV beams with 1 % energy spread, 1 mrad divergence, 1 µm size and 4 kA peak current. It is now installed at Laboratoire d’Optique Appliquée (LOA), where the LPA electrons are produced and accelerated. The divergence is rapidly mitigated (5 cm away from the source) via strong focusing with a triplet of so-called QUAPEVA permanent magnet quadrupoles of variable strength and of adjustable magnetic center position. A magnetic chicane then longitudinally stretches the beam, sorts electrons in energy and selects the energy range of interest via a removable and adjustable slit mounted in the middle of the chicane. A second set of quadrupoles matches the beam inside an in-vacuum undulator (typical SOLEIL 2 m long U20, cryo-ready U18 or 3 m long cryo-ready U15 prototype). The electron beam can be monitored with current transformers and cavity beam position monitors or by inserting scintillator screens along the line.
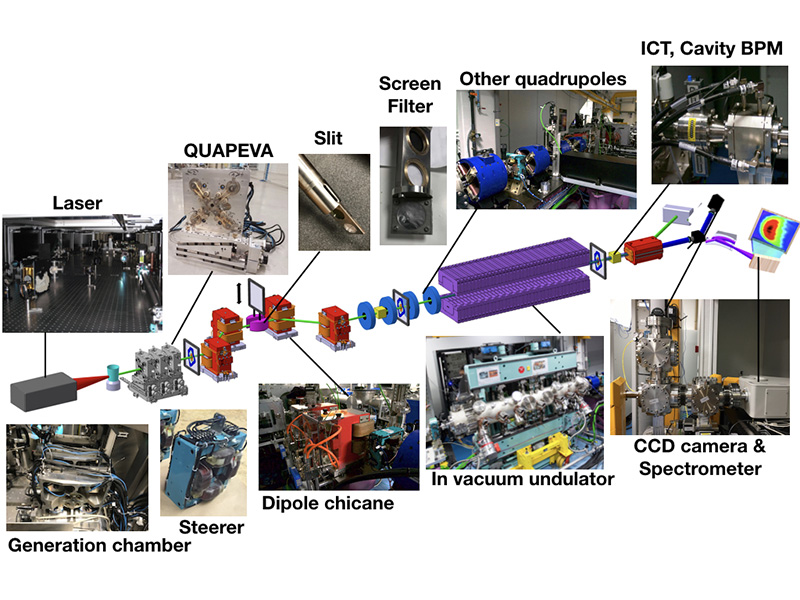
Figure 1: COXINEL line with its associated equipment
The LPA development (LOA) in the frame of an ERC Advanced Grant X-FIVE uses a Ti:Sapphire laser system delivering 1.5 J, 30 fs FWHM pulses. For the COXINEL experiment (see Fig. 2 of the installed line), the laser is focused into a supersonic jet of He-N2 gas mixture for the LPA to operate in the robust ionisation injection, providing beams of up to 100 pC in a broad energy range up to 200 MeV with 1-5 mrad divergence. Transfer line components and LPA laser are aligned within ± 100 μm on the same reference axis.
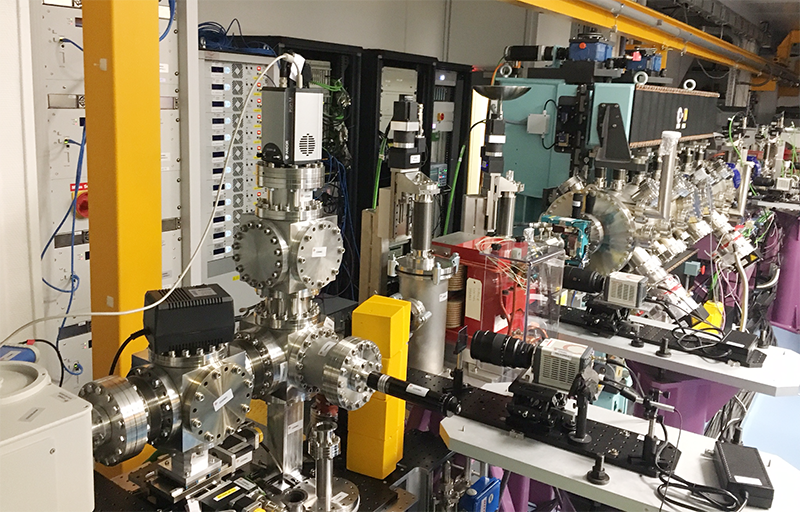
Figure 2: Picture of the installed COXINEL line
After a first rough beam transport along the line where chromatic effects play an important role, a Beam Position Alignment Compensation strategy based on the matrix response approach is developed for separate correction of beam dispersion and position thanks to a proper setting of the QUAPEVA magnetic axis, enabling mitigation of pointing fluctuations. The QUAPEVA strength is then slightly adjusted to optimize the focusing. The matched transported beam measurements agree with simulations for measured beam characteristics (dipole spectrometer and observation on a screen), as displayed in Fig. 3.
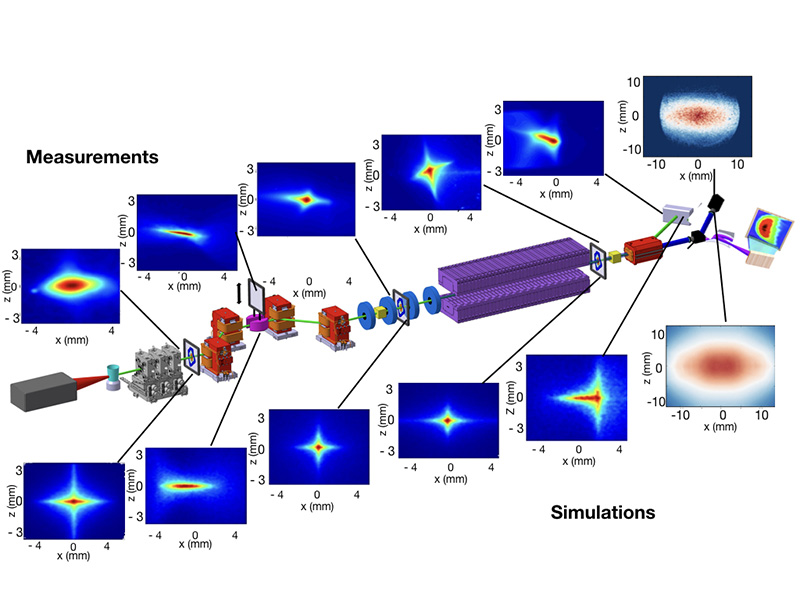
Figure 3: COXINEL electron beam / photon measurements and simulations
A slit in the chicane selects the desired energy range. U18 undulator radiation is characterized with a CDD camera with some bandpass filters for spectral selection, and with a spectrometer equipped with a CCD camera providing a moon shape type profile enabling to provide some insight on the beam quality. The possibility to observe FEL amplification depends strongly on the LPA beam parameters that can be experimentally achieved, and the qualification of the LPA with a FEL application still remains a major present Graal.
In conclusion, the scientists have shown that the LPA electron beam properties can be manipulated through an adequate transport line, mitigating the performance that do not meet the one of state-of-the-art conventional accelerators for some-specific applications. These results pave the way for further merging of novel and conventional accelerator techniques towards future applications, such as the new generation of colliders, requiring stages of LPA accelerating modules or free electron laser applications, relying on more optimised LPA performance that are progressing very fast.