Recently, an experimental branch, dedicated to UV and VUV absorption spectroscopy in the gas phase, was designed and installed on the DÉSIRS beamline. This branch combines a unique ultrahigh-resolution spectrometer with a multi-purpose vacuum chamber, which can accommodate various sample environments, to simulate the conditions encountered in different regions of space.
The recent detection of gravitational waves may open a new path for astronomy in the future, but up to now, electromagnetic radiation is our main information carrier. It is the bedrock of our understanding of the universe, from solar system planets to interstellar medium and beyond. Thanks to state of the art satellite instrumentation, measurements in spectral ranges which were hardly accessible before because of terrestrial atmosphere absorption are nowadays available. It is the case for deep UV (so called VUV for Vacuum Ultra Violet, for wavelengths shorter than 200 nm), which is very abundant in space, like, for example, the important Lyman a radiation, produced by de-excitation of atomic hydrogen. VUV is involved in a rich photochemistry, in the gas phase or in icy grains, and can participate to the formation of complex molecules from very simple precursors.
In this astrophysical context, inter alia, an experimental branch, dedicated to UV and VUV absorption spectroscopy in the gas phase, was designed and installed on the DESIRS beamline. This branch combines a unique ultrahigh-resolution spectrometer with a multi-purpose vacuum chamber, which can accommodate various sample environments, to simulate the conditions encountered in different regions of space. Indeed, in astrophysics, it is often necessary to benchmark observations with laboratory data. Without these data, physical models developed to understand the different processes leading to the observed species remain incomplete. It is particularly the case in the VUV range, where electronic transitions follow a complex spectroscopy for which a fully quantic modelling is needed. Relaxation mechanisms of the excited state (such as dissociation, which can strongly affect the respective abundance and survival of the various species) have to be accounted for.
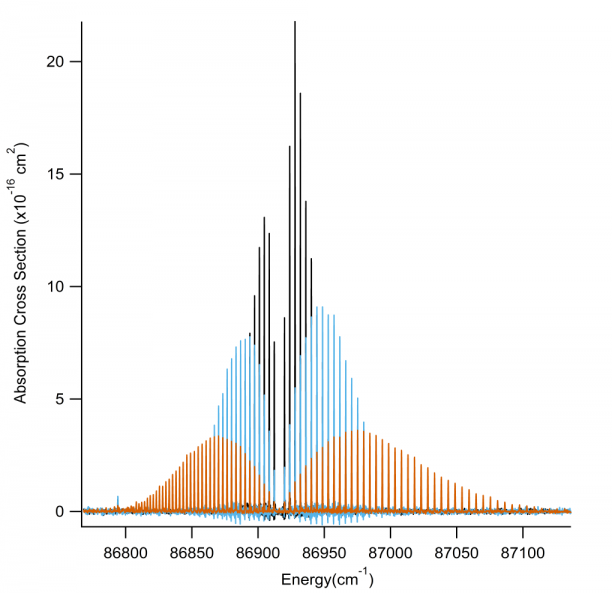
Figure 1: Absorption cross-sections for the CO molecule, around 115 nm and for different temperatures. Black: molecular jet (~48 K); Blue: windowless cell at room temperature; Orange: windowless cell at high temperature (~ 971 K). The resolving power of the DESIRS Fourier Transform spectrometer is high enough to observe the rotational structure of the molecule in the recorded spectra.
To understand, and in some cases simulate, this rich and complex photochemistry, it is important to be able to reproduce or to approach in laboratory the conditions (pressure, temperature) of various astrophysical environments. Several absorption cells were therefore developed on the Fourier Transform (FT) absorption spectroscopy branch of the DÉSIRS beamline. This end-station can now accommodate a liquid nitrogen cooled cell, a heated cell which can reach temperatures as high as 1000 K, and a molecular jet allowing to reduce the species rotational temperature down to approximately 10 K (figure 1). With these devices available to a broad user community, the experimental conditions can be tuned on the same sample quite easily. In particular, the set-up allows the determination of absolute absorption probabilities (cross sections), which are paramount parameters for models intending to predict the molecular composition and dynamics of interstellar space or planetary atmospheres. New set-ups are being developed, to study for example transient species like radicals, which are difficult to produce because of their reactivity (figure 2).
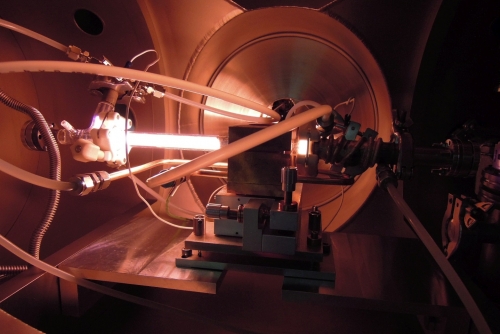
Figure 2: Transient species such as radicals produced in an electric discharge, can be probed by absorption spectroscopy with the VUV synchrotron radiation of the DESIRS beamline.