Metalloporphyrins are large molecules (fig. 1) whose cyclic structure includes a metal atom. Their role, essential in biological processes, depends mainly on the nature of the metal they contain: for example chlorophyll (photosynthesis) contains a porphyrin with magnesium; hemoglobin (oxygen transport) includes an iron porphyrin. Their varied electronic properties are of great interest for molecular electronics, chemical sensors or photovoltaics. This is why scientists are trying to control their synthesis.
Thanks to a specific equipment installed on the TEMPO beamline by Sorbonne University, researchers from the University of Trieste have shown that the presence of oxygen gas facilitates the porphyrin metalation step, which can thus take place at room temperature.
One of the crucial steps in the synthesis of a metalloporphyrin is the inclusion of the metal atom in the core of the ring (called tetrapyrrole) of the molecule.
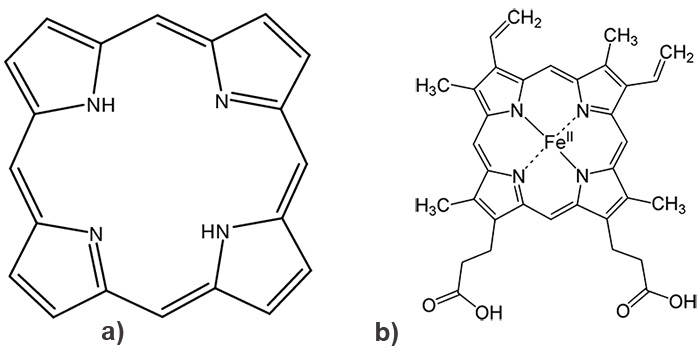
Figure 1:
a - structure of a porphyrin: cyclic macromolecule consisting of 4 pyrrole subunits joined together by bonds involving carbon atoms. Porphyrins can combine with metal atoms, linked at the central cavity of the molecule.
b - example of a metalloporphyrin: heme b, which is part of the composition of hemoglobin. The heme b hosts an Fe atom, in the ferrous form Fe2+ in the center of the structure, which allows the heme to fix the dioxygen O2 and to transport the oxygen in the blood.
In Nature, this reaction is catalyzed by enzymes, called chelatases, and takes place at room temperature. In the laboratory, the adsorption of a porphyrin on a metallic surface allows the molecule to be placed in the conditions necessary for metalation: metallic atoms from the surface can then diffuse into the molecule and associate with it by self-assembly; this is called self-metalation. However, this is a reaction that can have a high activation energy, which represents an obstacle. In Nature, the role of the catalyst (i.e. the enzyme) is to reduce this energy barrier so that the reaction becomes possible. But in the laboratory, other means must be found to lower this energy barrier. One of them consists in reaching an intermediate state of reaction –in which the porphyrin molecule becomes more likely to bind a metal atom– by oxidizing the surface on which the porphyrin is adsorbed. Then, one just need to increase the temperature so that the activation barrier is overcome, and the metalation of the porphyrin can occur. But the high temperatures required can damage the molecule.
Within the framework of a collaboration between the Physics Department of the University of Trieste, the CNR-IOM institute, and the associated TEMPO beamline team, it was shown that the self-metalation of hydrogenated tetraphenylporphyrin molecules (or "2H-TPP", a synthetic compound resembling natural porphyrin) adsorbed on a Palladium surface (Pd(100) ; Figure 2) can be promoted by oxygen gas, under temperature and pressure conditions close to ambient conditions. The gas-molecule-surface system resembles the configuration existing in the catalytic pocket of the enzyme where and when the reaction takes place in Nature.
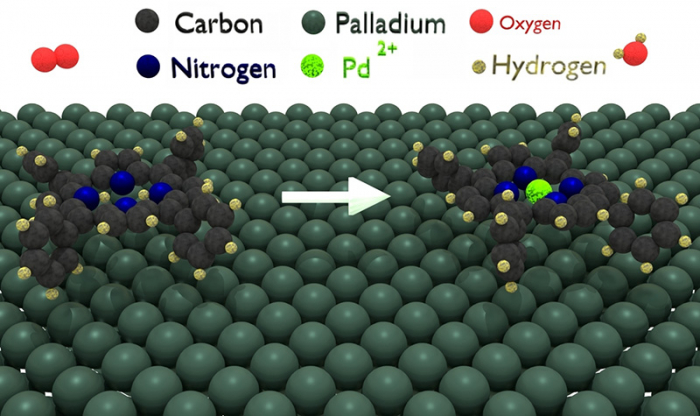
Figure 2: Schematic representation of tetraphenylporphyrin molecules adsorbed on the Palladium surface, in the presence of oxygen gas promoting metalation.
This result was demonstrated by a unique combination of vibrational spectroscopy (called infrared-visible sum frequency generation, or IR-Vis SFG) carried out at the University of Trieste and Near-Ambient Pressure X-Ray Photoelectron Spectroscopy (NAP-XPS) experiments on the TEMPO beamline.
It was shown that self-metalation can be induced by gas phase oxygen already at room temperature. The measured activation energy barrier is only 0.37-0.4 eV. The pyrrolic hydrogen atoms react via the catalytic mediation of a Pd atom, thus forming molecular hydrogen (Figure 3). The same pyrrolic hydrogen atoms in the center of the molecule can be transferred to an oxygen atom, again via the catalytic mediation of a Pd atom, thus forming water. An alternative route involves the Pd(100) surface, which accommodates the H atoms after metalation and before desorption of the metalloporphyrin.
However, computational results and experimental data show that the activation barriers depend on the surface and on the metal bound: the energy barriers span from zero or close, up to 1.5 eV. In this study, the value obtained (0.4 eV) lays in the lowest part of the range, thus supporting accessibility of this novel metalation route already at room temperature.
This result allows to consider new processes of metallization of organic structures on surfaces and, in the case of metalloporphyrins, could lead to the synthesis of molecules used for example for photocatalysis (conversion of solar energy), the production of hydrogen from water (water splitting), the design of biosensors ....
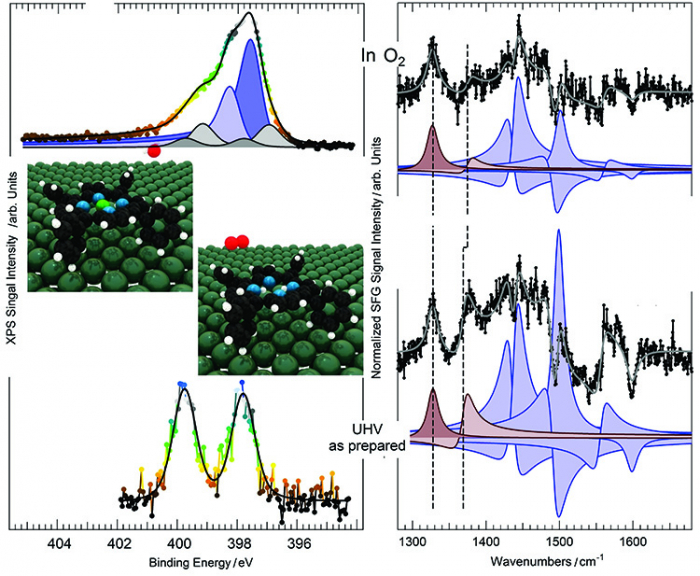
Figure 3:
Left: NAP-XPS spectra. The recorded signals are produced by the nitrogen atoms in the center of the 2H-TPP porphyrin adsorbed on the Pd(100) surface.
Bottom: 2H-TPP before metalation. The low binding energy peak corresponds to the nitrogen atoms bound to a hydrogen, and the other to the nitrogen atoms not bound to a hydrogen.
Top: 2H-TPP after metalation. The spectrum has changed drastically. The main peak corresponds to the N atoms bound to the Pd atom included by the metalation process; the peak due to the nitrogen atoms bound to a hydrogen has disappeared. The lower intensity peaks correspond to by-products.
Right: IR-Vis SFG spectra. They show the different vibrations of the molecule. The vibrations indicated by the vertical dashed lines involve the nitrogen atoms and witness the self-metalation induced by oxygen.